Musculoskeletal Pathology Discussion-Functional Anatomy & Physiology
TABLE OF
CONTENTS
1 Embryology of the Musculoskeletal
System. 2
2 Arthrology. 2
3 Pain. 11
4 Assessment of Musculoskeletal
Disorders. 14
5 Concepts of Management 14
6 Peripheral Joint Mobilization
Techniques. 14
7 Automobilization Techniques for
the Extremities. 14
8 Friction Massage. 14
9 Relaxation. 22
10 Shoulder and Shoulder Girdle. 22
11 Elbow.. 29
12 Wrist and Hand Complex. 31
13 Hip. 35
14 Knee. 36
15 Ankle and Hindfoot 38
16 Temporomandibular Joint 137
17 Vertebral Column. 137
18 Spine-General Structure and
Biomechanical Considerations Kessler 139
19 Cervical Spine Kessler 139
20 Lumbar Spine Kessler 139
2.1
2.2
Kinematics
2.2.1
Classification of Joint Surfaces and Movements
2.2.2
Joint Surfaces
Back Table of Contents References
2.2.3.1.1 The neutral or zero position for measuring
joint motion is as follows.
2.2.3.1.1.1 Erect posture
2.2.3.1.1.2 Face forward
2.2.3.1.1.3 Palms of hands forward
2.2.3.1.1.4 Fingers and thumbs in extension
2.2.3.1.1.5 Feet flat on the floor
2.2.3.1.2 The sections below on planes and axes will
use this position as reference.
2.2.3.2.1 An axis is a straight line about which the
joint rotates. The three basic axes described below are at right angles to each
other.
2.2.3.2.1.1
Sagittal axis
2.2.3.2.1.1.1
Placed on the
Sagittal plane it runs horizontally from anterior to posterior. The movements
of abduction and adduction occur around this axis in a coronal plane.
2.2.3.2.1.2 Coronal (Frontal) Axis
2.2.3.2.1.2.1
A horizontal
line in the coronal plane situated from side to side. Flexion and extension
occur about this axis in the Sagittal plane.
2.2.3.2.1.3 Longitudinal Axis
2.2.3.2.1.3.1
This is a
vertical line extending in a superior –inferior direction. Medial and lateral
rotation, and horizontal abduction and adduction occur in the transverse plane.
2.2.3.2.1.4 Exceptions
2.2.3.2.1.4.1
These
exceptions are explained in the sections for the thumb and scapula.
Back Table of Contents References
2.2.3.3.1 The three basic planes of reference are
derived from the dimensions in space and are at right angles to each other.
2.2.3.3.2 Sagittal Plane
2.2.3.3.2.1 The Sagittal plane is vertical and extends
from front to back, deriving its name from the direction of the Sagittal suture
of the skull. It may also be called an anterior-posterior plane. The median
Sagittal plane, midsagittal, divides the body into right and left halves.
2.2.3.3.3 Coronal (Frontal) Plane
2.2.3.3.3.1 The coronal plane is vertical and extends
from side to side, deriving its name from the direction of the coronal suture
of the skull. It is also called the frontal or lateral plane, and divides the
body into an anterior and a posterior portion.
2.2.3.3.4 Transverse (Horizontal) Plane
2.2.3.3.4.1 A transverse plane is horizontal and
divides the body into upper (cranial) and lower (caudal) portions
2.2.3.4.1 The point at which the three midplanes of
the body intersect is the center of gravity which in an ideally aligned posture
in a so-called average adult human being, slightly anterior to the first or
second sacral segment.
2.2.3.5.1 The line of gravity is a vertical line
through the center of gravity
2.2.4
Joint Movements
Back Table of Contents References
2.2.4.1.1.1
Coronal Axis
2.2.4.1.1.1.1
2.2.4.1.1.2
Flexion
2.2.4.1.1.2.1
2.2.4.1.1.3
Extension
2.2.4.1.1.3.1
2.2.4.1.1.4
Embryonic
Development
2.2.4.1.1.4.1
Back Table
of Contents References
2.2.4.1.2.1
2.2.4.1.3.1
Sagittal Axis
2.2.4.1.3.1.1
2.2.4.1.3.2
Abduction and
Adduction
2.2.4.1.3.2.1
2.2.4.1.4.1
2.2.4.1.5.1
2.2.4.1.6.1
Back Table of Contents References
2.2.4.1.7.1 Longitudinal Axis
2.2.4.1.7.1.1
2.2.4.1.7.2 Lower Extremities
2.2.4.1.7.2.1
2.2.4.1.7.3 Upper Extremities
2.2.4.1.7.3.1
2.2.4.1.8.1
2.2.4.1.8.2 Head and Pelvis
Anterior and Posterior Tilt
2.2.4.1.8.2.1
2.2.4.1.8.3
Head and
Pelvis Lateral Tilt
2.2.4.1.8.3.1
2.2.4.1.8.4
Scapula
2.2.4.1.8.4.1
2.2.4.2
Movements of Specific Joints
2.2.4.2.1 Metacarpophalangeal
Joints of Fingers
2.2.4.2.1.1 Abduction and
Adduction
2.2.4.2.1.1.1 Occurs
about a Sagittal axis. The line of reference for abduction and adduction of the
fingers is the axial line through the third digit.
2.2.4.2.1.2 Abduction
2.2.4.2.1.2.1 Abduction
is movement in the plane of the palm away from the axial line, spreading
fingers wide apart. The third digit may move in abduction both ulnarly and
radially from the axial line.
2.2.4.2.1.2.2 Movement
in the plane of the palm away from a axial line of reference through the third
metacarpal & digit
2.2.4.2.1.2.3 Simply
movement away from the third finger
2.2.4.2.1.3 Adduction
2.2.4.2.1.3.1 Adduction
is movement in the plane of the palm toward the axial line, that is, closing
the extended fingers together sideways.
2.2.4.2.1.4 Circumduction
2.2.4.2.1.4.1 Circumduction
is the combination of flexion, abduction, extension, and adduction movements
performed consecutively, in either direction, at the Metacarpophalangeal joints
of the fingers. Extension in these condyloid joints is somewhat limited so that
the base of the cone described by the fingertip is relatively small.
2.2.4.2.2 Ankle
Joint
2.2.4.2.2.1 The ankle joint is
a ginglymus or hinge joint formed by the articulation of the tibia and fibula
with the talus. The axis about which motion takes place extends obliquely from the
posterolateral aspect of the fibular malleolus to the Anteromedial aspect of
the tibial malleolus.
2.2.4.2.2.2 Flexion and
Extension
2.2.4.2.2.2.1 These
movements occur about the oblique axis. Flexion (plantarflexion) is movement of
the foot in which the plantar surface moves in a caudal and posterior
direction. Extension (dorsiflexion) is movement of the foot in which the dorsal
surface moves in an anterior and cranial direction.
2.2.4.2.2.2.2
The knee should be flexed when measuring dorsiflexion. With
the knee flexed, the ankle joint can be dorsiflexed about 20°. If the knee is
extended, the gastrocnemius will limit the range of motion to about 10° of
dorsiflexion. The range of motion in plantar flexion is approximately 45°.
2.2.4.2.3 Subtalar
Joint (Talocalcaneonavicular)
2.2.4.2.3.1 The subtalar joint
is a modified plane or gliding joint formed by the articulation of the talus
and the calcaneus. The talus also articulates with the navicular, and the
talonavicular joint is involved in the movements ascribed to the subtalar
joint.
2.2.4.2.3.2 Supination and
Pronation
2.2.4.2.3.2.1 Movements
permitted by the subtalar and talocalcaneonavicular joints. Supination is
rotation of the foot in which the sole of the foot moves in a medial direction;
pronation is rotation in which the sole of the foot moves in a lateral
direction.
2.2.4.2.4 Transverse
Tarsal Joints
2.2.4.2.4.1 The transverse
tarsal joints are formed by the articulations of the talus with the navicular,
and the calcaneus with the cuboid.
2.2.4.2.4.2 Adduction and
Abduction
2.2.4.2.4.2.1
Movements of the forefoot permitted by the transverse tarsal
joints, adduction is movement of the forefoot in a medial direction and
abduction is movement in a lateral direction.
2.2.4.2.4.3 Inversion
2.2.4.2.4.3.1 A
combination of supination and forefoot adduction. It is more free in plantar
flexion than in dorsiflexion.
2.2.4.2.4.3.2 Rotation
of the foot and movement of the forefoot in a medial direction
2.2.4.2.4.4 Eversion
2.2.4.2.4.4.1
A combination of pronation and forefoot abduction. It is more
free in dorsiflexion than in plantar flexion.
2.2.4.2.4.4.2
Rotation of the foot and movement of the forefoot in a lateral
direction
2.2.4.2.5 Metatarsophalangeal
Joints
2.2.4.2.5.1 The
metatarsophalangeal joints are condyloid, formed by the articulation of the
distal ends of the metatarsals with the adjacent ends of the proximal
phalanges.
2.2.4.2.5.2 Flexion and
extension
2.2.4.2.5.2.1
Movements about a coronal axis. Flexion is movement in a
caudal direction, extension is movement in a cranial direction. The range of
motion in adults is variable, but 30°
flexion and 40° extension may be considered an average range for good
function of the toes.
2.2.4.2.5.3 Adduction and
Abduction
2.2.4.2.5.3.1
Movements about a sagittal axis. The line of reference for
adduction and abduction of the toes is the axial line projected distally in
line with the second metatarsal and extending through the second digit.
2.2.4.2.5.3.2
Abduction is movement away from a Sagittal line of reference
through the second metatarsal & digit.
2.2.4.2.5.3.3
Simply abduction is movement away from the second toe.
2.2.4.2.5.3.4
Adduction is movement toward the axial line, and abduction is
movement away from it, as in spreading the toes apart. Because abduction of the
toes is restricted by the wearing of shoes, this movement is markedly limited
in most adults and little attention is paid to the ability to abduct.
2.2.4.2.5.4 Interphalangeal
Joints of toes
2.2.4.2.5.4.1
The Interphalangeal joints are ginglymus or hinge joints
formed by the articulations of adjacent surfaces of phalanges.
2.2.4.2.5.4.2
Flexion and extension are movements about a coronal axis with flexion
being movement in a caudal direction and extension movement in a cranial
direction.
2.2.5
Arthrokinematics
2.2.6
Joint Play
2.2.7
Conjunct rotation
2.2.8
Summary of Joint Function
2.3
Clinical Applications
2.3.1
Terminology
2.3.2
Analysis of Accessory Joint Motions
2.4
Neurology
2.4.1
2.4.2
Innervation
2.4.3
Receptors
2.4.3.1
2.4.3.2
Type 1
2.4.3.3
Type 2
2.4.3.4
Type 3
2.4.3.5
Type 4
2.4.4
Clinical Considerations
2.5
Joint Nutrition
2.6
Lubrication
2.6.1
2.6.2
Models of Joint Lubrication
2.6.3
Resolving Problems of Joint-Surface
Wear
2.7
Approach to Management of Joint
Dysfunction
2.7.1
Pathologic Considerations
2.7.1.1
Increased Rate of Tissue Breakdown
2.7.1.2
Reduced Rate of Tissue Breakdown
2.7.1.3
Increased Rate of Tissue Production
2.7.1.4
Reduced Rate of Tissue Production and
Repair
2.7.1.5
Intervention and Communication
2.7.1.6
Arthrosis
2.7.1.7
The Degenerative Cycle
2.7.1.8
Capsular Tightness
2.7.1.9
Joint Effusion
2.7.1.10 Relative Capsular Fibrosis
2.7.1.11 Clinical Considerations
3.1 Melzack-Wall Gate Theory AK Synopsis 259
3.1.1 Melzack and Wall[1]
[2]
state that nerve impulses are brought from the receptors to both large fibers
and small fibers. These two types of fibers have different characteristics.
3.1.1.1
Large Fibers
3.1.1.1.1 Myelinated
A Fibers
3.1.1.1.2 Fast
conduction (up to 120 Meters/second)
3.1.1.1.3 Have
receptors that react to low and moderate intensity stimuli
3.1.1.2
Small Fibers
3.1.1.2.1 Unmyelinated
C Fibers
3.1.1.2.2 Slow
conduction (down to 1 meter/second)
3.1.1.2.3 Have
receptors that react to low, moderate, and high intensity stimuli
3.1.2 When there is a stimulus to a receptor field, both large and small
fibers conduct impulses. As the stimulation becomes noxious (such as a pain
stimulus), the small fibers conduct with greater intensity due to their
receptors’ characteristic reaction to high intensity stimuli.
3.1.3 The large and small fibers activate the transmission cells (T
cells), which project the information to the brain. The T cells are located in
the spinal cord dorsal horns, apparently in lamina 5. They fire when a certain
threshold of stimulation is reached.
3.1.4 The large diameter fibers and the small diameter fibers give off
branches to the substantia gelatinosa. The substantia gelatinosa is located in
the dorsal horn lamina 2 and 3. As a functional unit it extends the length of
the spinal cord on each side. Its cells connect with one another by short
fibers; they influence each other at distant sites on the same side by means of
Lissauer’s tract, and on the opposite side by means of commissural fibers that
cross the cord. The substantia gelatinosa therefore receives afferent input
from large and small fibers. Its cells connect with one another to different
levels of the spinal cord and they communicate with the contralateral side. The
spinal gate mechanism appears to be at the substantia gelatinosa.
3.1.5 Activity in the large fibers stimulates the substantia gelatinosa;
activity of the small fibers inhibits it; Activity of the substantia gelatinosa
inhibits activity of the T cell. Thus the balance of activity between the large
and small fibers either activates or deactivates the substantia gelatinosa
which, in turn, either allows activity at the T cell of inhibits activity
there. When the T cell is inhibited, information received by the cell cannot be
transmitted to the brain.
3.1.6 Adaptation to mild and moderate stimuli, primarily conducted by the
large nerve fibers, is accomplished by this gate mechanism. For example, when
you sit down in a chair to read a book, your nervous system soon adapts to the
pressure of sitting in the chair, your hand lying in your lap, and the pressure
of the book in your hand. These mild pressure stimuli are conducted primarily
by the large fibers to both the substantia gelatinosa and the T cells. Upon
first conduction, the T cells transmit information to the brain, which is
interpreted as pressure. With continued stimulation, the substantia gelatinosa
sends impulses of an inhibiting nature to the T cells. This reduces the
information about the sitting position, which is being transmitted to the
brain, so the body (large fibers) adapts to the stimulation. If, on the other
hand, you sit on a sharp tack, there would not only be transmission by the
large fibers but also increased transmission by the small fibers, which are
activated more by high intensity stimulation. The activity of the small fibers
stimulates the substantia gelatinosa and the AT cells. The immediate response
is transmission by the T cells to the brain, informing it of the noxious
stimuli. The continued inhibitory stimulation of the substantia gelatinosa
would turn its activity off, giving no inhibitory action to the T cells;
consequently, the T cells would continue to transmit information of a pain
stimulus to the brain. This is the manner in which the gate is held open by
pain stimuli
3.1.7 Continued sitting on the tack and the subsequent transmission of the
T cells trigger the action system required by the pain. The activity will be as
follows;
3.1.7.1
Perceptual information, giving
location, magnitude, and spatiotemporal properties of the noxious stimulus
3.1.7.2
Motivational tendency toward escape or
attack
3.1.7.3
Cognitive information based on
analysis of past experience and probable outcome of different response
strategies.
3.1.8 The interplay of these three activities could then influence motor
mechanisms responsible for the complex pattern of overt responses that
characterize pain.
3.1.9 Also added to the model is the central control mechanism. By this
mechanism central activities, such as anxiety or excitement, may open or close
the gate for inputs from any part of the body. The central control cortical
projections and reticular projections explain how higher central nervous system
processes- such as attention, anxiety, anticipation, and past experience- exert
a powerful influence on pain processes.
8
Friction Massage
8.1 Introduction
8.1.1 A particularly important massage technique in the management of
many, musculoskeletal disorders is deep transverse friction massage. Its
importance and the rationale and technique of application have not been well
described in the traditional literature.
8.1.2 Many of the chronic musculoskeletal disorders seen clinically are
manifestations of the body's response to the fatigue stresses. Tissues tend to respond to fatigue stresses
by increasing the rate of tissue production.
Thus, prolonged abnormal stresses to a tissue will lead to tissue
hypertrophy, provided that the nutritional status of the tissue is not
compromised and that the stress rate (the rate of tissue breakdown) does not
exceed the rate at which this tissue can repair the microdamage. Under continuing stress, if nutrition to the
tissue is affected or if the rate of tissue breakdown is excessive, the tissue
will gradually atrophy and weaken to the point of eventual failure. Tissues that normally have a low metabolic
rate (usually those that are relatively poorly vascularized) are most
susceptible to such degeneration. Such
tissues include articular cartilage, inter-articulate fibrocartilage, tendons,
and some ligaments. On the other hand,
those tissues with good vascularity and a normally high rate of turnover, such
as cancellous bone, muscle, capsular tissue, and some ligaments, are more likely
to respond by undergoing hypertrophy.
This results in increased density of the structural elements. Of course, even the structures may not be
able to keep up with the rate of tissue breakdown is the stress rate is two or
under conditions of reduced nutrition (E.G., hypovascularity)
8.1.3 Under conditions of mildly increased stress rates, the body has the
ability to adapt adequately, and no pathological state (i.e. pain,
inflammation, or dysfunction) results.
Such conditions might even include situations of high magnitude stresses
if the high stress levels are induced gradually and the stresses are
intermittent enough to allow an interval for adequate repair to take
place. A typical example is the
individual engaging in vigorous athletic activities who goes through a period
of gradual training. The training
period allows for adequate maturation of new tissue so that structural elements
become oriented in ways that best attenuate energy without yielding. Such energy attenuation requires that there
be a sufficient mass of tissue to provide some resistance to deformation, but
it also requires that the structure be adequately extensible to minimize the
strain on individual structural elements.
To increase the ability of a structure to attenuate the energy of work
done on it (a force tending to deform the structure), new collagen is produced
to increase the tissues total ability to resist the force. However, this new collagen must be
sufficiently mobile to permit some deformation. The less it deforms, the greater the resistance the tissue must
offer. The greater the resistance it must offer, the greater will be the
internal strain on individual collagen fibers or bony trabeculae. The greater the strain on individual structural
elements, the greater the rate of microdamage.
As the rate of microdamage increases, so does likelihood of pain and
inflammation. As you can see, a more
massive tissue is not necessarily one that will permit normal functioning under
increased stress. It must also be
deformable, and deformability requires time for the new structural elements
(collagen fibers and bony trabeculae) to assume the proper "weave."
8.1.4 The effects of the weave, or orientation, a structural elements in
contributing to the extensibility of a structure as a whole can be appreciated
by examining a Chinese "finger trap". You can lengthen and shorten the finger trap without changing the
length of any of the individual fibers composing it. Its extensibility is due entirely to the weave of the fibers and
interfiber mobility. Thus, you can
apply an extending force to the structure without inducing internal strain on
any of the individual fibers. If the
fibers were not in the proper weave or if they were to stick to one another,
the deforming force would be met with greater resistance by the structure and
greater internal strain to individual fibers.
The body adapts to mildly increased stress rates by laying down collagen
precursors, which, in response to imposed stresses, polymerize into collagen
fibers. The fibers become oriented in
the proper weave to allow deformability of the tissue.
8.1.5 Under abnormally high stress levels or altered nutritional
conditions, the body’s ability to adapt may be inadequate. The particular structure may not be able to
produce new tissue fast enough, or the new tissue that is produced may not have
sufficient time or proper inducement to mature. In the former situation, the tissue will degenerate, whereas in
the latter, pain and inflammation are likely to result if stresses
continue. Tissue degeneration must be
treated by reducing stress levels and/or increasing nutrition to the tissue,
depending on the underlying cause.
Typical examples of such tissue degeneration would include the
degradation of articular cartilage in degenerative joint disease, and the
lesions that commonly affect the soft tissues of the diabetic foot. Articular
cartilage, being avascular and having a normally low metabolic turnover, does
not adapt well to increased stress levels and is thus susceptible to the
fatigue degeneration. The diabetic foot
may have a nutritional deficit because of vascular changes and possibly
increased stresses secondary to reduced sensory feedback, leaving it abnormally
susceptible to tissue breakdown.
8.1.6 Situations in which the new tissue does not mature adequately are
typically those in which the stress levels are not sufficient to cause
degeneration but are too excessive to allow time for normal tissue
modelling. In bone, the condition is
referred to as sclerosis; in capsules, ligaments, and tendons, in may be
referred to as fibrosis. In both
situations there is often a normal or increased amount of tissue, but the
tissue is not sufficiently deformable to attenuate the energy of loading from
use of the part. This can cause pain, inflammation, and/or increased stresses
to adjacent tissues. Correction of such
conditions requires that stress levels be reduced while stresses sufficient to
stimulate normal tissue modelling are maintained. In addition, normal extensibility of the structure must be restored. This requires that interfiber mobility be
increased. The nutritional status of
the tissue must also be considered.
8.1.7 There are many common musculoskeletal disorders that may be related
to abnormal or inadequate tissue modelling.
Bony sclerosis typically occurs in degenerative joint disease when there
are abnormal compressive stresses to a joint.
Most tendonitis can be attributed to continued abnormal stresses to a
tendon, which preclude adequate tissue modelling and create a structure that is
not sufficiently deformable. This is
especially true of the condition often referred to as tennis elbow, in which
the origins of the extensor carpi radialis brevis becomes fibrosed, and a
chronic inflammatory process arises.
Rotator cuff tendonitis, usually involving the supraspinatus or
infraspinatus regions of the tendinous cuff, is a very common disorder in which
normal modelling is compromised by hypovascularity to the area of
involvement. Often these lesions at the
shoulder progress to stage at which gradual degeneration and eventual failure
ensue. It is likely that the capsular
fibrosis associated with "frozen shoulder" is a similar disorder of
tissue modelling; the joint capsule hypertrophies in response to increase
stress levels, but in doing so loses its extensibility. Abnormal tissue modelling will also result
when a tissue is immobilized during the repair phase of an inflammatory
process. Thus, a fracture may "heal,"
but normal modelling bony trabecula requires resumption of normal stress
levels. Similarly, a joint capsule will
become fibrosed when the joint is immobilized following arthrotomy; collagen is
laid down in response to the traumatic inflammatory process affecting the
synovium, but the lack of movement permits in an organized network of fibers
that forms abnormal interfiber bonds (adhesions) that do not extend normally
when the part is moved.
8.1.8 The approach to treatment of conditions in which continued stresses
have not allowed the structure to mature adequately must include measures to reduce
stresses to the part. We must consider
means of reducing loading of the part as well as means of preventing excessive
internal strain. Reduced loading might
be accomplished through control of activities, the use of orthotic devices to
control alignment or movement, or the use of assistive devices such as
crutches. Also, to reduce loading of a
particular tissue, the capacity of other tissues to attenuate more of the
energy of loading might be increased.
Increasing the strength and activities of related muscles often does
this. Thus, if we wish to reduce the likelihood of excessive loading of the
anterior talofibular ligament, the peroneal muscles should be
strengthened. However, we can also
strap the ankle to provide additional afferent input to reflexively enhance the
ability of the peroneals to contract.
8.1.9 Reduction in stress levels alone, however, will not assure that
adequate maturation will take place. As
mentioned earlier, stress to the part is a necessary stimulus for the
restoration of normal alignment of structural elements. This apparent paradox is understood when we
consider that reducing stress is necessary in order to allow new tissue to be
laid down and reconstituted, while at the same time some stress is necessary to
optimise the nutritional status of the part and to effect proper orientation
and mobility of the new tissue.
Consequently, in the case of most chronic musculoskeletal disorders,
resolution is not likely to take place with either complete rest of the part or
unrestricted use. A judgment must be
made, then, as to the appropriate activity level for a particular disorder and
the rate at which normal activities can be resumed. This judgment must be based on data gained from an examination
that reflects the nature and extent of the pathologic process as well as
etiologic considerations. Knowledge of
the healing responses of musculoskeletal tissues and of their responses to
various stress conditions must also be applied.
8.1.10
In situations in which significant
reduction of activities is necessary in order to allow healing to current,
there are measures that the therapist can, and should take. The practitioner must help prevent undue
dysfunction that may result from a mass of tissue being laid down as
unorganized, adherent cicatrix, and from the atrophy of related muscle groups
that is likely to take place. There a
few conditions, even of an acute inflammatory nature, in which some gentle
range of motion and isometric muscle exercises cannot be performed during the
healing process without detrimental effects.
8.1.11
Some of the chronic disorders that can
to be the most persistent are minor lesions of tendons and ligaments. These are often refractory to treatments
such as rest and anti-inflammatory therapy because they are not chronic inflammatory
lesions per se, but pathological processes resulting from abnormal modelling of
tissue in response to fatigue stresses.
Therefore, while rest allows new tissue to be produced, that which is
produced is not of normal extensibility because of lack of a proper orientation
of structural elements, abnormal adherence of structural elements to one
another, and adherent to adjacent tissues.
In some situations, most notably rotator cuff tendonitis, inadequate
tissue nutrition is also a factor.
Because the lack of extensibility that accompanies "healing"
of these lesions, the structure becomes more susceptible to internal strain
when stresses are resumed and less able to attenuate the energy of loads
applied to it. The result is recurrence
of a low-grade inflammatory process each time use of the part is resumed. The most common these disorders are
supraspinatus tendonitis at the shoulder, tendonitis of the origin of the
extensor carpi radialis brevis (tennis elbow), tendonitis of the abductor
pollicis longus or extensor pollicis brevis tendons that the wrist (de
Quervain’s disease), coronary ligament sprain at the knee, and anterior
talofibular ligament sprain.
8.1.12
In such chronic, persistent lesions of
tendons and ligaments- and occasionally muscle- procedures to promote normal
mobility and extensibility of the involved structure are important components
of the treatment program. Passive or
active exercises that impose a longitudinal strain on the involved structure
may be incorporated. However, this
creates the risk of maintaining the weakened or unresolved state of healing by
contributing to the rate of tissue micro damage. That is probably why these disorders can not to resolve
spontaneously with varying degrees of activity. Too little activity results in loss of extensibility; too much
activity does not allow for adequate healing.
The appropriate compromise is difficult to judge.
8.1.13
Another method of promoting increased
extensibility and mobility of the structure, while reducing stress levels and
allowing healing to take place, is the use of deep transverse friction
massage. This is a form of treatment
advocated primarily Cyriax, but unfortunately not widely adopted to date. It involves applying a deep massage directly
to the site of the lesion in an direction perpendicular to normal orientation
of fibrous elements. This maintains
mobility of the structure with respect to adjacent tissues and probably helps
to promote increased interfiber mobility of the structure itself without
longitudinally stressing it. It may
also promote normal orientation of fibers as they are produced. This effect might be likened to be effective
rolling your hand over and unorganized pile of toothpicks; eventually the
toothpicks will all become oriented perpendicular the direction in which the
hand moves. In some pathological
processes, such as rotator cuff tendonitis, in which the etiology may be
related to the nutritional deficit arising from hypovascularity, the hyperemia
induced by the friction massage may also contribute to the healing
response.
8.1.14
Although these effects of friction
massage are highly conjectural, they're based on sound physiological and
pathological concepts. Further support
is provided by the often dramatically favorable results obtained clinically
when friction massage is appropriately incorporated in a treatment
program. Studies are needed, however,
to substantiate the physiological effects and clinical efficacy of friction
massage in these chronic disorders.
Designing a legitimate clinical study would be difficult because most of
the disorders for which friction massage seems to be effective do not present
with measurable objective signs, and documentation of subjective improvement is
usually unreliable. Basic studies of
the effects of friction massage, however may be fashioned after previous
investigations into the effects of exercise, immobility, and other variables on
the healing and maturation of collagen tissue.
Until we have more concrete evidence of the value for should massage
succumb its use must be justified on in the considerations combined with
"educated empiricism."
10.1
Review of Functional Anatomy
Back Table
of Contents References
10.1.1.1 Articulations
10.1.1.1.1
Clavicle
10.1.1.1.1.1
10.1.1.1.2
Scapula
10.1.1.1.2.1
10.1.1.2
Joint Motion
10.1.1.2.1
Sternoclavicular
Joint
10.1.1.2.1.1
10.1.1.3 Scapular Position
10.1.1.3.1
10.1.1.4 Muscular Attachments
10.1.1.4.1
10.1.1.5
Basic Movements
10.1.1.5.1
Adduction
10.1.1.5.1.1
10.1.1.5.2
Abduction
10.1.1.5.2.1
10.1.1.5.3
Lateral
or Upward Rotation
10.1.1.5.3.1
10.1.1.5.4
Medial
or Downward Rotation
10.1.1.5.4.1
10.1.1.5.5
Anterior
Tilt
10.1.1.5.5.1
10.1.1.5.6
Elevation
10.1.1.5.6.1
10.1.1.5.7
Depression
10.1.1.5.7.1
10.1.1.5.8
Note
10.1.1.5.8.1
Back Table of Contents References
10.1.2.1
Kendall
10.1.2.1.1
Introduction
10.1.2.1.1.1
Type
of Joint
10.1.2.1.1.1.1
Synovial
10.1.2.1.1.1.2
Spheroid
(Ball & Socket)
10.1.2.1.1.2
The
head of the humerus and the glenoid cavity of the scapula form the Glenohumeral
joint.
10.1.2.1.2
Flexion
and Extension
10.1.2.1.2.1
Plane
of Movement
10.1.2.1.2.1.1
Sagittal
10.1.2.1.2.2
Axis
of Movement
10.1.2.1.2.2.1
Coronal
10.1.2.1.2.3
Range
of Movement (Flexion)
10.1.2.1.2.3.1
225°
10.1.2.1.2.3.2
The
arc of movement along the Sagittal plane begins at 45° extension forward
through the zero anatomical position and on to the 180° overhead position.
10.1.2.1.2.3.3
Other
Joint Involvement
10.1.2.1.2.3.3.1
Scapular
10.1.2.1.2.3.3.2
Glenohumeral
flexion accounts for only about 120° and the remaining 60° is achieved as a
result of abduction and lateral rotation of the scapula. The scapula moves in
this way to face the head of the humerus anteriorly to provide for its stable
placement in the joint cavity as it moves thru its arc to a fully vertical position.
10.1.2.1.2.3.3.3
The
scapular motion is at first variable but remains constant after about 60°
10.1.2.1.2.3.3.4
Inman
et al.[3]
observed that between 30° and 170° flexion the Glenohumeral joint moved 10° and
the scapula rotated 5° for every 15° of motion.
10.1.2.1.2.4
Range
of Movement (Extension)
Back Table of Contents References
10.1.2.1.2.4.1
225°
10.1.2.1.2.4.2
The
arc of movement along the Sagittal plane begins at 180° flexion backward
through the zero anatomical position and on to the 45° to the extended
position.
10.1.2.1.2.4.3
If the
elbow joint is flexed the range of shoulder joint extension will be increased
because the tension of the biceps will be released.
10.1.2.1.3
Abduction
and Adduction
10.1.2.1.3.1
10.1.2.1.3.2
Abduction
10.1.2.1.3.2.1
10.1.2.1.3.3
Adduction
10.1.2.1.3.3.1
10.1.2.1.4
Horizontal
Abduction and Adduction
10.1.2.1.4.1
10.1.2.1.4.2
Horizontal
Abduction
10.1.2.1.4.2.1
10.1.2.1.4.3
Horizontal
Adduction
10.1.2.1.4.3.1
10.1.2.1.5
Medial
and Lateral Rotation
10.1.2.1.5.1
10.1.2.1.5.2
Medial
Rotation
10.1.2.1.5.2.1
10.1.2.1.5.3
Lateral
Rotation
10.1.2.1.5.3.1
10.1.2.1.6
Circumduction
10.1.2.1.6.1
10.1.3
Osseous Structures Kessler 169
10.1.3.1 Glenohumeral Joint
10.1.3.1.1
Spinal
stability is essential for the adequate functioning of the shoulder girdle
complex, which includes the following osseous structures.
10.1.3.1.1.1
Upper
thoracic vertebrae
10.1.3.1.1.2
1st
and 2nd ribs
10.1.3.1.1.3
Manubrium
10.1.3.1.1.4
Scapula
10.1.3.1.1.5
Clavicle
10.1.3.1.1.6
Humerus
10.1.3.1.2
Full
Arm Elevation Essentials
10.1.3.1.2.1
Full
arm elevation can only be achieved when the upper thoracic vertebrae is able to
do the following on the ipsilateral side.
10.1.3.1.2.1.1
Extend
10.1.3.1.2.1.2
Rotate
10.1.3.1.2.1.3
Sidebend
10.1.3.1.2.2
The
first and second ribs must be able to descend and move posteriorly (with
vertebral rotation).
10.1.3.1.2.3
The following
joint structures must permit the Manubrium to sidebend and rotate to the
ipsilateral side.
10.1.3.1.2.3.1
Manubriosternal
10.1.3.1.2.3.2
Costomanubrial
10.1.3.1.2.3.3
Sternoclavicular
10.1.3.1.2.4
Mobility
of the scapulothoracic mechanism is dependant of the mobility of the following
joints.
10.1.3.1.2.4.1
Acromioclavicular
10.1.3.1.2.4.2
Sternoclavicular
10.1.3.1.2.5
The
Glenohumeral joint moves between 90° (active) and 120° (passive) elevation.
10.1.3.1.2.6
Full
arm elevation is accomplished in concert with the movement of the following
structures.
10.1.3.1.2.6.1
Scapular
rotation
10.1.3.1.2.6.2
Clavicular
elevation
10.1.3.1.2.6.3
Thoracic
extension
10.1.3.1.2.7
The
lower thoracic vertebrae must sidebend away from the side of motion.
10.1.3.1.2.8
Exaggeration
of lumbar Lordosis must accompany full arm elevation and is achieved by the
action of the spinal muscles.[4]
10.1.3.2 Acromioclavicular Joint
10.1.3.3 Sternoclavicular Joint
10.1.3.4 Scapulothoracic Mechanism
10.1.4
Ligaments
10.1.4.1 Glenohumeral Joint
10.1.4.2 Acromioclavicular Joint
10.1.4.3 Sternoclavicular Joint
10.1.5
Bursae
10.1.5.1
10.1.5.2 Subacromial or Subdeltoid Bursa
10.1.5.3 Subscapular Bursa
10.1.6
Vascular Anatomy of Rotator Cuff
Tendons
10.1.6.1
10.2
Biomechanics
10.2.1
Joint Stabilization
10.2.2
Influence of the Glenohumeral Joint Capsule
on Movement
10.2.3
Muscular Force Couple
10.2.4
Analysis of Shoulder Abduction
Back Table of Contents References
11.1.1.1 Type of Joint
11.1.1.1.1
11.1.1.2 Movements Permitted
11.1.1.2.1
11.1.1.3 Description
11.1.1.3.1
11.1.2
Flexion
11.1.2.1
11.1.3
Extension
11.1.3.1
Back Table of Contents References
11.2.1
Type of Joint
11.2.1.1
11.2.2
Movements Permitted
11.2.2.1
11.2.3
Description
11.2.3.1
11.2.4
Supination
and Pronation
11.2.4.1
Supination
11.2.4.1.1
11.2.4.2
Pronation
11.2.4.2.1
11.2.4.3
Shoulder
Rotation
11.2.4.3.1
11.2.4.4
Neutral
or Zero Position
11.2.4.4.1
Back Table of Contents References
12.1.1
12.1.2
Flexion
and Extension
12.1.2.1
12.1.2.2
Flexion
12.1.2.2.1
12.1.2.3
Extension
12.1.2.3.1
12.1.3
Abduction
(Radial Deviation) and Adduction
12.1.3.1
12.1.3.2
Adduction
12.1.3.2.1
12.1.3.3
Abduction
12.1.3.3.1
12.1.4
Circumduction
12.1.4.1
Back Table of Contents References
12.2.1.1
Type of Joint
12.2.1.1.1
12.2.1.2
Movements Permitted
12.2.1.2.1
12.2.1.3
Description
12.2.1.3.1
12.2.2.1
Type of Joint
12.2.2.1.1
12.2.2.2
Movements Permitted
12.2.2.2.1
12.2.2.3
Description
12.2.2.3.1
12.2.2.4
Flexion
and Extension
12.2.2.4.1
12.2.2.4.2
Flexion
12.2.2.4.2.1
12.2.2.4.3
Extension
12.2.2.4.3.1
12.2.2.5
Abduction
and Adduction
12.2.2.5.1
12.2.2.5.2
Abduction
12.2.2.5.2.1
12.2.2.5.3
Adduction
12.2.2.5.3.1
12.2.2.6
Circumduction
12.2.2.6.1
Back Table of Contents References
12.2.3.1
Type of Joint
12.2.3.1.1
12.2.3.2
Movements Permitted
12.2.3.2.1
12.2.3.3
Description
12.2.3.3.1
12.2.4.1
Type of Joint
12.2.4.1.1
12.2.4.2
Movements Permitted
12.2.4.2.1
12.2.4.3
Description
12.2.4.3.1
12.2.4.4
Adduction and Abduction
12.2.4.4.1
12.2.4.4.2
Adduction
12.2.4.4.2.1
12.2.4.4.3
Abduction
12.2.4.4.3.1
12.2.4.5
Rotation
12.2.4.5.1
12.2.4.6
Opposition
12.2.4.6.1
12.2.4.6.2
Muscles
12.2.4.6.2.1
12.2.4.7
Circumduction
12.2.4.7.1
Back Table of Contents References
12.2.5.1
Type of Joint
12.2.5.1.1
12.2.5.2
Movements Permitted
12.2.5.2.1
12.2.5.3
Description
12.2.5.3.1
12.2.5.4
Flexion and Extension
12.2.5.4.1
12.2.5.4.2
Flexion
12.2.5.4.2.1
12.2.5.4.3
Extension
12.2.5.4.3.1
12.2.5.5
Abduction, Adduction, and Rotation
12.2.5.5.1
Back Table of Contents References
13.1.1.1 Neutral Position
13.1.1.1.1
13.1.1.2 Anterior Pelvic Tilt
13.1.1.2.1
13.1.1.3 Posterior Pelvic Tilt
13.1.1.3.1
13.1.1.4 Lateral Pelvic Tilt
13.1.1.4.1
13.2.1.1 Type of Joint
13.2.1.1.1
13.2.1.2 Movements Permitted
13.2.1.2.1
13.2.1.3 Range of Movement
13.2.1.3.1
13.2.1.4 Description
13.2.1.4.1
13.2.1.5 Flexion and Extension
13.2.1.5.1
13.2.1.5.2
Flexion
13.2.1.5.2.1
13.2.1.5.3
Extension
13.2.1.5.3.1
13.2.1.6 Abduction and Adduction
Back Table of Contents References
13.2.1.6.1
13.2.1.6.2
Abduction
13.2.1.6.2.1
13.2.1.6.3
Adduction
13.2.1.6.3.1
13.2.1.7 Lateral and Medial Rotation
13.2.1.7.1
13.2.1.7.2
Medial Rotation
13.2.1.7.2.1
13.2.1.7.3
Lateral Rotation
13.2.1.7.3.1
Back Table of Contents References
14.1.1.1 Type of Joint
14.1.1.1.1
14.1.1.2 Movements Permitted
14.1.1.2.1
14.1.1.3 Range of Movement
14.1.1.3.1
14.1.1.4 Description
14.1.1.4.1
14.1.1.5 Flexion and Extension
14.1.1.5.1
14.1.1.5.2
Flexion
14.1.1.5.2.1
14.1.1.5.3
Extension
14.1.1.5.3.1
14.1.1.6 Hyperextension
14.1.1.6.1
14.1.1.7 Lateral and Medial Rotation
14.1.1.7.1
14.1.1.7.2
15.1 Neurology
15.1.1
15.2 Osteology
15.2.1
Introduction
15.2.1.1 The tibia flares at its distal end. As a result, the cross section
of the bone changes from triangular, in the region of the shaft,
to quadrangular in the area of the distal metaphyseal portion of the bone.
Medially there is a distal projection of the tibia, the medial malleolus;
located laterally is the fibular notch, which is concave anteroposteriorly for
articulation with the distal end of the fibula. Along the medial side of the
posterior surface is a groove for the passage of the tibialis posterior tendon.
The term posterior malleolus .is often used to refer to the distal
overhang of the posterior aspect of the tibia (Fig. 15-1).
15.2.1.2
The lateral surface of the medial
malleolus and the inferior surface of the tibia have a continuous cartilaginous
covering for articulation with the talus. The articular surface of the inferior
end of the tibia is concave anteroposteriorly. Mediolaterally, it is somewhat
convex, having a crest centrally that corresponds to the central groove in
.the trochlear surface of the talus. This, then, is essentially a sellar joint
surface. It is slightly wider anteriorly than posteriorly. The articular
surface of the medial malleolus is comma shaped with the "tail" of
the comma being situated posteriorly (see Fig. 15 -1). .
15.2.1.3 The fibula, which is quite narrow
in the region of its shaft, becomes bulbous at its distal end (Fig. 152). This
distal portion of the bone, the lateral malleolus, is triangular in cross
section. When viewed from a lateral aspect, the fibula is somewhat pointed
distally. The lateral malleolus extends farther distally and is situated more
posteriorly than the medial malleolus. The medial aspect of the lateral
malleolus is covered by a triangular cartilaginous surface for articulation with the lateral side of the talus. Above this surface,
the fibula contacts the tibia in the fibular notch of the tibia. The apex of this
triangular surface points inferiorly. There is a fairly deep depression in the
posterior inferior region of the lateral malleolus termed the malleolar
fossa .that can be easily palpated. The posterior talofibular ligament
attaches in this fossa. There is a groove along the posterior aspect of the
lateral malleolus through which the peroneus brevis tendon passes.
15.2.1.4 The talus constitutes the link between the leg and the tarsus (Fig.
15 - 3). It consists of a body, anterior. to which is, the head, The body and
head of the talus are connected by a short neck.
15.2.1.5 The superior surface- of the body of the talus is covered with
articular cartilage for articulation with the inferior surface of the tibia.
This articular surface is continuous with the articular surfaces of the medial
and lateral aspects of the talus. The superior surface is somewhat wider
anteriorly than posteriorly, It is convex anteroposteriorly and slightly
concave mediolaterally, corresponding to the sellar surface of the inferior end
of the tibia mentioned previously. In this sense, the superior talar articular
surface is trochlear, or pulley-like, and is often referred to as .the
trochlea.
15.2.1.6 The lateral aspect of the body of the talus is largely covered by articular
cartilage for articulation with the distal end of the fibula (Fig. 15 -4). This
articular_ surface is triangular, with the apex situated inferiorly. Just
below this apex is a lateral bony projection to which the lateral talocalcaneal
ligament attaches.
15.2.1.7 The articular surface of the medial aspect of the talus is
considerably smaller than that of the lateral side, and it faces slightly
forward (Fig. 15 -5,) It. contacts the articular surface of the medial
malleolus on the tibia. It is comma shaped, with the tail of the comma being
situated posteriorly. The roughened area below the medial articular surface
serves as an attachment for the deltoid ligament. The medial and lateral talar
articular surfaces tend to converge posteriorly, leading to the wedge shape of
the trochlea. It should be emphasized, however, that the lateral articular
surface of the talus is perpendicular to the axis of movement at the ankle
joint, whereas the medial surface is not. This has important biomechanical
implications, which are discussed in the following section.
15.2.1.8 If one views the profiles of
the lateral and medial sides of the trochlea, the lateral profile is seen as a
section of a circle, whereas the medial profile may be viewed as sections of
several circles of different radii; the medial profile is of smaller radius
anteriorly than posteriorly.[5]
More precisely stated, the contour medially is of gradually increasing radius
anteroposteriorly, forming a cardioid profile. The importance of this is
described in the section on biomechanics.
15.2.1.9
Posteriorly the body of the talus is
largely covered by a continuation of the trochlear articular surface as it slopes backward (Fig. 15 - 6).
At the inferior extent of the posterior aspect is the nonarticular posterior
process. The posterior process consists of a lateral and a smaller medial
tubercle, with an intervening groove through which passes the tension of the
flexor hallucis longus.
15.2.1.10
Figure
15-1
15.2.1.10.1
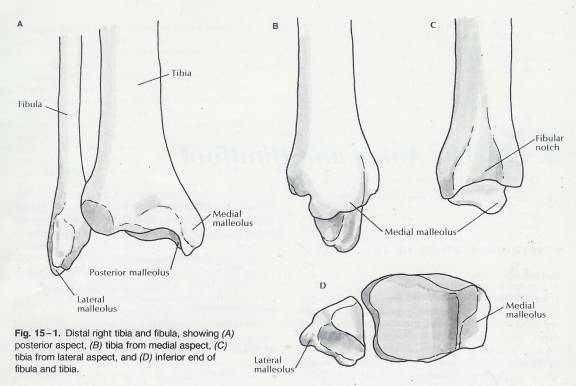
15.2.1.11
Figure
15-2
15.2.1.11.1
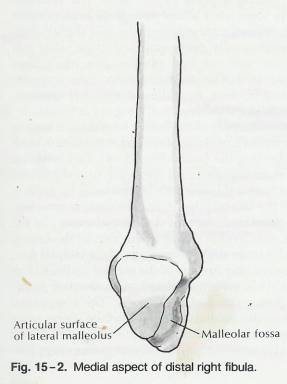
15.2.1.12
Figure
15-3 & 15-4
15.2.1.12.1
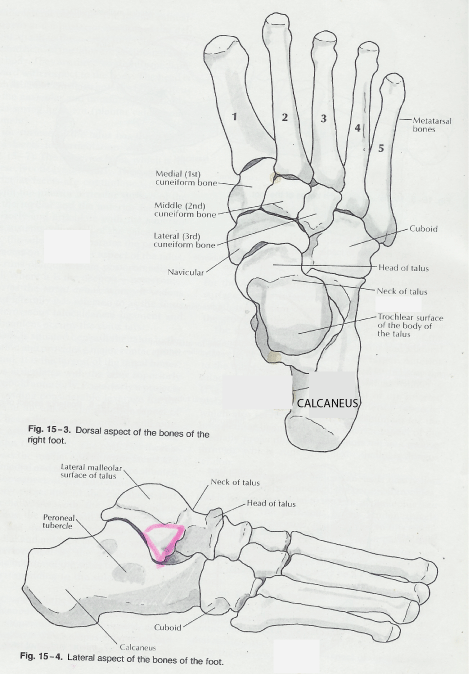
15.2.1.13
Figure
15-5, 6, & 7
15.2.1.13.1
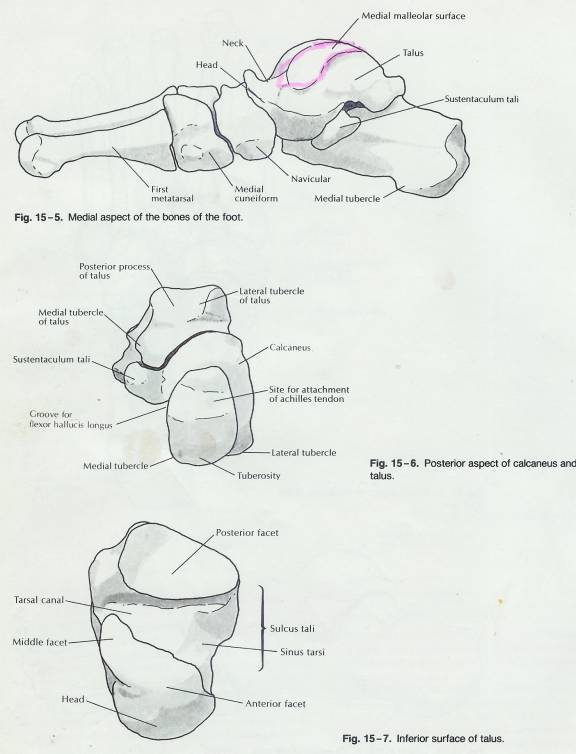
15.2.1.14 The posterior talofibular ligament attaches to the 1ateral tubercle. The medial talocalcaneal ligament
and a posterior portion of the deltoid ligament attach to the medial tubercle.
15.2.1.15
The neck and head of the talus are
positioned anteriorly to the body. They are directed slightly medially and
downward with respect to the body. The head is covered with articular cartilage
anteriorly for articulation with the navicular and inferiorly for articulation
with the spring ligament (plantar calcaneonavicular ligament.
15.2.1.16 The
inferior: surface of the talus has three cartilage-covered facets for
articulation with the calcaneus (Fig. 15 -7). The posterior facet, which is the
largest of these, is concave inferiorly. The medial and anterior articular
facets are continuous with each other-and with the inferior articular surface
of the head. Both the medial and the anterior facets are convex inferiorly and
articulate with the superior aspect of the sustentaculum tali of the calcaneus.
A deep groove, the sulcus tali, separates the posterior and medial facets on
the inferior aspect of the talus. This groove runs obliquely from posteromedial
to anterolateral. Where it is the deepest-posteromedially-it forms the tarsal
canal; where it widens and opens out laterally it is referred to as the sinus.
Tars. The interosseous talocalcaneal ligament and the cervical ligament
occupy the sinus tarsi.
15.2.1.17 The
calcaneus is situated beneath the talus in the standing position and provides a
major contact point with the ground. It is the largest of the tarsal bones. The
calcaneus articulates with the talus superiorly and with the cuboid anteriorly.
Posteriorly it projects backward, providing considerable leverage for the
plantar flexors of the ankle. The superior aspect of the calcaneus bears the
posterior, medial, and anterior facets for articulation with the corresponding
facets of the talus (Fig. 15 - 8). The posterior facet is convex whereas the
medial and anterior facets are concave. The medial and anterior facets are
situated on the superior aspect of the sustentaculum tali, which is a bony
projection of the calcaneus that overhangs medially. As with the corresponding
facets on the talus, the medial and anterior facets of the calcaneus are
usually continuous with each other. The medial and anterior facets are
separated' from the posterior facet by the sulcus calcanei, which forms
the bottom of the sinus tarsi and tarsal canal, thus corresponding to the
sulcus tali of the talus.
15.2.1.18 The
posterior aspect of the large posterior projection of the calcaneus contains a
smooth superior surface, which slopes upward and forward, and a rough inferior
surface, which slopes downward and forward.
15.2.1.19
Figure
15-8
15.2.1.19.1
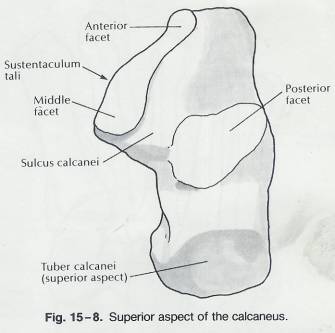
15.2.1.20
The upper surface is the site of
attachment for the Achilles tendon (see Fig. 15 - 6). The lower surface Blends
inferiorly with the tuber calcanei, which is the point of contact of the
calcaneus with the
ground in the standing position.
15.2.1.21 The
tuber calcanei on the inferior aspect of the" calcaneus consists of a
medial tubercle and a lateral tubercle, of which the is the larger. Anterior to
the tuber calcanei is a roughened surface for the attachment of the long and
short plantar ligaments (Fig. 15-9). At the anterior extent of the inferior
surface of the calcaneus is the anterior tubercle, which also serves as a point
of attachment for the long plantar ligament. On the inferior aspect of the
medially projecting sustentaculum tali is a groove through which runs the
flexor hallucis .longus tendon.
15.2.1.22 The
lateral aspect of the calcaneus is nearly flat. There is a small prominence,
the peroneal trochlea that is located just distal to the lateral malleolus (see
Fig. 15 - 4). The peroneus brevis tendon travels downward and forward, just
superior to this trochlea: while the peroneus longus tendon passes inferior to
it. The calcaneofibular ligament attaches just posterior and slightly superior
to the peroneal trochlea, at which point there may be a rounded prominence.
15.2.1.23 From
the anterosuperior extent of the medial aspect of the calcaneus, the
sustentaculum tali projects in a medial direction (see Fig. 15-8) The sustentaculum
tali may be palpated just below the medial malleolus. .
15.2.1.24
Figure
15-9
15.2.1.24.1
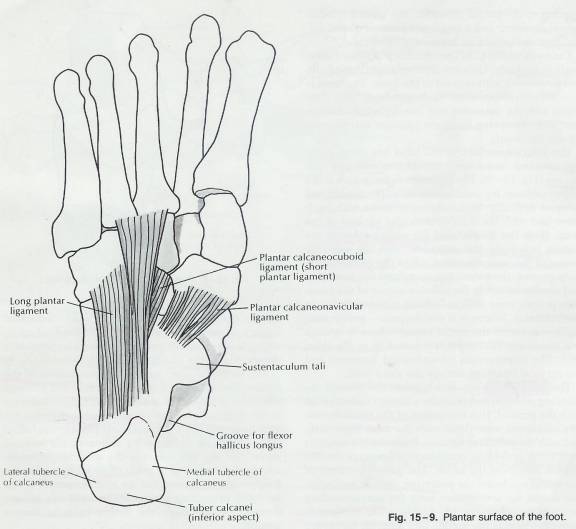
15.2.1.25 On
the narrowed anterior aspect of the calcaneus is the cartage-covered articular
surface. that contacts the cuboid bone. This is a sellar joint surface, being
concave, superoinferiorly and convex mediolaterally (see Fig. 15-3).
15.2.1.26 The
remainder of the tarsus includes the navicular and cuboid bones, which contact
the talus and calcaneus, respectively, and the three cuneiforms, which
articulate with the first three metatarsals (Fig. 15-3). The cuboid extends
distally to contact the remaining two metatarsals. (These bones will not be
considered in detail here but will be referred to in the biomechanics section
of this chapter).
15.2.2
Tarsals,
Metatarsals, and Phalanges
15.2.2.1
Tarsus
(Tarsals) (7) (tahr’sus)
15.2.2.1.1
The tarsus is
a collective designation for the seven bones of the ankle called tarsals. The
term tarsos pertains to a broad, flat surface.
15.2.2.1.2
Posterior
Tarsus
15.2.2.1.2.1
Talus (astragalus; ankle bone)
15.2.2.1.2.1.1
Summary
15.2.2.1.2.1.1.1
The talus, the uppermost
tarsal bone, is the only bone of the foot that articulates with the fibula and
tibia.
15.2.2.1.2.1.1.2
It is
surrounded on one side by the medial Malleolus of the tibia and on the other
side by the lateral Malleolus of the fibula.
15.2.2.1.2.1.1.3
During walking,
the talus initially bears the entire weight of the body. About half the weight
is then transmitted to the calcaneus. The remainder is transmitted to the other
tarsal bones.
15.2.2.1.2.1.2
Detailed
Description of Osseous Structure
15.2.2.1.2.1.2.1
The talus is
the second largest of the tarsal bones. It occupies the middle and upper part
of the tarsus, supporting the tibia above, resting upon the calcaneus below,
articulating on either side with the malleoli, and in front with the navicular.
It consists of a body, a neck, and a head.
15.2.2.1.2.1.2.2
The Body
(corpus tali)
15.2.2.1.2.1.2.2.1
The superior surface of the body presents, behind, a smooth
trochlear surface, the trochlea, for articulation with the tibia. The trochlea
is broader in front than behind, convex from before backward, slightly concave
from side to side: in front it is continuous with the upper surface of the neck
of the bone. The inferior surface
presents two articular areas, the posterior and middle calcaneal surfaces,
separated from one another by a deep groove, the sulcus tali. The groove runs
obliquely forward and lateralward, becoming gradually broader and deeper in
front: in the articulated foot it lies above a similar groove upon the upper
surface of the calcaneus, and forms, with it, a canal (sinus tarsi) filled up
in the fresh state by the interosseous talocalcaneal ligament. The posterior
calcaneal articular surface is large and of an oval or oblong form. It
articulates with the corresponding facet on the upper surface of the calcaneus,
and is deeply concave in the direction of its long axis which runs forward and
lateralward at an angle of about 45° with the median plane of the body. The
middle calcaneal articular surface is small, oval in form and slightly convex;
it articulates with the upper surface of the sustentaculum tali of the
calcaneus. The medial surface presents at its upper part a pear-shaped
articular facet for the medial malleolus, continuous above with the trochlea;
below the articular surface is a rough depression for the attachment of the
deep portion of the deltoid ligament of the ankle-joint. The lateral surface
carries a large triangular facet, concave from above downward, for articulation
with the lateral malleolus; its anterior half is continuous above with the
trochlea; and in front of it is a rough depression for the attachment of the
anterior talofibular ligament. Between the posterior half of the lateral border
of the trochlea and the posterior part of the base of the fibular articular
surface is a triangular facet, which comes into contact with the transverse
inferior tibiofibular ligament during flexion of the ankle-joint; below the
base of this facet is a groove, which affords attachment to the posterior
talofibular ligament.
15.2.2.1.2.1.2.2.2
The posterior surface is narrow, and
traversed by a groove running obliquely downward and medialward, and transmitting
the tendon of the Flexor hallucis longus. Lateral to the groove is a prominent
tubercle, the posterior process, to which the posterior talofibular ligament is
attached; this process is sometimes separated from the rest of the talus, and
is then known as the os trigonum. Medial to the groove is a second smaller
tubercle.
15.2.2.1.2.1.2.3
The Neck
(collum tali)
15.2.2.1.2.1.2.3.1
The neck is directed forward and medialward, and comprises the
constricted portion of the bone between the body and the oval head. Its upper
and medial surfaces are rough, for the attachment of ligaments; its lateral
surface is concave and is continuous below with the deep groove for the
interosseous talocalcaneal ligament.
15.2.2.1.2.1.2.4
The Head (caput tali)
15.2.2.1.2.1.2.4.1
The head looks forward and medialward; its anterior articular
or navicular surface is large, oval, and convex. Its inferior surface has two
facets, which are best seen in the fresh condition. The medial, situated in
front of the middle calcaneal facet, is convex, triangular, or semi-oval in
shape, and rests on the plantar calcaneonavicular ligament; the lateral, named
the anterior calcaneal articular surface, is somewhat flattened, and
articulates with the facet on the upper surface of the anterior part of the
calcaneus.
15.2.2.1.2.1.2.5
Articulations.
15.2.2.1.2.1.2.5.1
The talus articulates with four bones: tibia, fibula,
calcaneus, and navicular.
15.2.2.1.2.2
Calcaneus (kal-KĀ-nē-us) (os calcis)
15.2.2.1.2.2.1
Summary
15.2.2.1.2.2.1.1
The calcaneus,
or heel bone, is the largest and strongest tarsal bone.
15.2.2.1.2.2.2
Detailed
Description of Osseous Structure
15.2.2.1.2.2.2.1
Introduction
15.2.2.1.2.2.2.1.1
The calcaneus is the largest of the tarsal bones. It is
situated at the lower and back part of the foot, serving to transmit the weight
of the body to the ground, and forming a strong lever for the muscles of the
calf. It is irregularly cuboidal in form, having its long axis directed forward
and lateralward; it presents for examination six surfaces.
15.2.2.1.2.2.2.2
Surfaces
15.2.2.1.2.2.2.2.1
Superior Surface
1.1.1.1.1.1.1.1.1
The superior surface extends behind on to that part of the
bone which projects backward to form the heel. This varies in length in
different individuals, is convex from side to side, concave from before
backward, and supports a mass of fat placed in front of the tendo calcaneus. In
front of this area is a large usually somewhat oval-shaped facet, the posterior
articular surface, which looks upward and forward; it is convex from behind
forward, and articulates with the posterior calcaneal facet on the under
surface of the talus. It is bounded anteriorly by a deep depression which is
continued backward and medialward in the form of a groove, the calcaneal
sulcus. In the articulated foot this sulcus lies below a similar one on the
under surface of the talus, and the two form a canal (sinus tarsi) for the
lodgment of the interosseous talocalcaneal ligament. In front and to the medial
side of this groove is an elongated facet, concave from behind forward, and
with its long axis directed forward and lateralward. This facet is frequently
divided into two by a notch: of the two, the posterior, and larger is termed
the middle articular surface; it is supported on a projecting process of bone,
the sustentaculum tali, and articulates with the middle calcaneal facet on the
under surface of the talus; the anterior articular surface is placed on the
anterior part of the body, and articulates with the anterior calcaneal facet on
the talus. The upper surface, anterior and lateral to the facets, is rough for
the attachment of ligaments and for the origin of the Extensor digitorum
brevis.
15.2.2.1.2.2.2.2.2
Inferior or Plantar surface
1.1.1.1.1.1.1.1.2
The inferior or plantar surface is uneven, wider behind than in
front, and convex from side to side; it is bounded posteriorly by a transverse
elevation, the calcaneal tuberosity, which is depressed in the middle and
prolonged at either end into a process; the lateral process, small, prominent,
and rounded, gives origin to part of the Abductor digiti quinti; the medial
process, broader and larger, gives attachment, by its prominent medial margin,
to the Abductor hallucis, and in front to the Flexor digitorum brevis and the
plantar aponeurosis; the depression between the processes gives origin to the
Abductor digiti quinti. The rough surface in front of the processes gives
attachment to the long plantar ligament, and to the lateral head of the
Quadratus plantae while to a prominent tubercle nearer the anterior part of this
surface, as well as to a transverse groove in front of the tubercle, is
attached the plantar calcaneocuboid ligament. The lateral surface is broad
behind and narrow in front, flat and almost subcutaneous; near its center is a
tubercle, for the attachment of the calcaneofibular ligament. At its upper and
anterior part, this surface gives attachment to the lateral talocalcaneal
ligament; and in front of the tubercle it presents a narrow surface marked by
two oblique grooves. The grooves are separated by an elevated ridge, or
tubercle, the trochlear process (peroneal tubercle), which varies much in size
in different bones. The superior groove transmits the tendon of the Peroneus
brevis; the inferior groove, that of the Peroneus longus.
15.2.2.1.2.2.2.2.3
Medial Surface
1.1.1.1.1.1.1.1.3
The medial surface is deeply concave; it is directed obliquely
downward and forward, and serves for the transmission of the plantar vessels
and nerves into the sole of the foot; it affords origin to part of the
Quadratus plantae. At its upper and forepart is a horizontal eminence, the
sustentaculum tali, which gives attachment to a slip of the tendon of the
Tibialis posterior. This eminence is concave above, and articulates with the
middle calcaneal articular surface of the talus; below, it is grooved for the tendon
of the Flexor hallucis longus; its anterior margin gives attachment to the
plantar calcaneonavicular ligament, and its medial, to a part of the deltoid
ligament of the ankle-joint. The anterior or cuboid articular surface is of a
somewhat triangular form. It is concave from above downward and lateralward,
and convex in a direction at right angles to this. Its medial border gives
attachment to the plantar calcaneonavicular ligament. The posterior surface is
prominent, convex, wider below than above, and divisible into three areas. The
lowest of these is rough, and covered by the fatty and fibrous tissue of the
heel; the middle, also rough, gives insertion to the tendo calcaneus and
Plantaris; while the highest is smooth, and is covered by a bursa which intervenes
between it and the tendo calcaneus.
15.2.2.1.2.2.2.2.4
Articulations
1.1.1.1.1.1.1.1.4
The calcaneus articulates with two bones: the talus and
cuboid.
15.2.2.1.3
Anterior
Tarsus
15.2.2.1.3.1
The anterior part contains the cuboid, Navicular, and three
cuneiform (cuneiform=wedge-shaped) bones called the first (medial), second
(intermediate), and third (lateral) cuneiform.
15.2.2.1.3.2
Cuboid Bone (os cuboideum)
15.2.2.1.3.2.1
Introduction
15.2.2.1.3.2.1.1
The cuboid bone is placed on the lateral side of the foot, in
front of the calcaneus, and behind the fourth and fifth metatarsal bones. It is
of a pyramidal shape, its base being directed medialward.
15.2.2.1.3.2.2
Surfaces
15.2.2.1.3.2.2.1
Dorsal Surface
15.2.2.1.3.2.2.1.1
The dorsal surface, directed upward and lateralward, is rough,
for the attachment of ligaments.
15.2.2.1.3.2.2.2
Plantar Surface
15.2.2.1.3.2.2.2.1
The plantar surface presents in front a deep groove, the
peroneal sulcus, which runs obliquely forward and medialward; it lodges the
tendon of the Peroneus longus, and is bounded behind by a prominent ridge, to
which the long plantar ligament is attached. The ridge ends laterally in an
eminence, the tuberosity, the surface of which presents an oval facet; on this
facet glides the sesamoid bone or cartilage frequently found in the tendon of
the Peroneus longus. The surface of bone behind the groove is rough, for the
attachment of the plantar calcaneocuboid ligament, a few fibers of the Flexor
hallucis brevis, and a fasciculus from the tendon of the Tibialis posterior.
15.2.2.1.3.2.2.3
Lateral Surface
15.2.2.1.3.2.2.3.1
The lateral surface presents a deep notch formed by the
commencement of the peroneal sulcus.
15.2.2.1.3.2.2.4
Posterior Surface
15.2.2.1.3.2.2.4.1
The posterior surface is smooth, triangular, and
concavo-convex, for articulation with the anterior surface of the calcaneus;
its inferior-medial angle projects backward as a process, which underlies and
supports the anterior end of the calcaneus.
15.2.2.1.3.2.2.5
Anterior Surface
15.2.2.1.3.2.2.5.1
The anterior surface, of smaller size, but also irregularly
triangular, is divided by a vertical ridge into two facets: the medial,
quadrilateral in form, articulates with the fourth metatarsal; the lateral,
larger and more triangular, articulates with the fifth.
15.2.2.1.3.2.2.6
Medial Surface
15.2.2.1.3.2.2.6.1
The medial surface is broad, irregularly quadrilateral, and
presents at its middle and upper part a smooth oval facet, for articulation
with the third cuneiform; and behind this (occasionally) a smaller facet, for
articulation with the navicular; it is rough in the rest of its extent, for the
attachment of strong interosseous ligaments.
15.2.2.1.3.2.2.7
Articulations
15.2.2.1.3.2.2.7.1
The cuboid articulates with four bones: the calcaneus,
third cuneiform, and fourth and fifth metatarsals; occasionally with a fifth,
the navicular.
15.2.2.1.3.3
Navicular Bone (os naviculare pedis; scaphoid bone)
15.2.2.1.3.3.1
Summary
15.2.2.1.3.3.1.1
The navicular bone is situated at the medial side of the
tarsus, between the talus behind and the cuneiform bones in front.
15.2.2.1.3.3.2
Surfaces
15.2.2.1.3.3.2.1
Anterior Surface
15.2.2.1.3.3.2.1.1
The anterior surface is convex from side to side, and subdivided
by two ridges into three facets, for articulation with the three cuneiform
bones.
15.2.2.1.3.3.2.2
Posterior Surface
15.2.2.1.3.3.2.2.1
The posterior surface is oval, concave, broader laterally than
medially, and articulates with the rounded head of the talus.
15.2.2.1.3.3.2.3
Dorsal Surface
15.2.2.1.3.3.2.3.1
The dorsal surface is convex from side to side, and rough for
the attachment of ligaments.
15.2.2.1.3.3.2.4
Plantar Surface
15.2.2.1.3.3.2.4.1
The plantar surface is irregular, and also rough for the
attachment of ligaments.
15.2.2.1.3.3.2.5
Medial Surface
15.2.2.1.3.3.2.5.1
The medial surface presents a rounded tuberosity, the lower
part of which gives attachment to part of the tendon of the Tibialis posterior.
15.2.2.1.3.3.2.6
Lateral Surface
15.2.2.1.3.3.2.6.1
The lateral surface is rough and irregular for the attachment
of ligaments, and occasionally presents a small facet for articulation with the
cuboid bone.
15.2.2.1.3.3.3
Articulations
15.2.2.1.3.3.3.1
The navicular articulates with four bones: the talus and the
three cuneiforms; occasionally with a fifth, the cuboid.
15.2.2.1.3.4
The First Cuneiform Bone (os cuneiform primum;
internalcuneiform)
15.2.2.1.3.4.1
Summary
15.2.2.1.3.4.1.1
The first cuneiform bone is the largest of the three
cuneiforms. It is situated at the medial side of the foot, between the
navicular behind and the base of the first metatarsal in front.
15.2.2.1.3.4.2
Surfaces
15.2.2.1.3.4.2.1
Medial Surface
15.2.2.1.3.4.2.1.1
The medial surface is subcutaneous, broad, and quadrilateral;
at its anterior plantar angle is a smooth oval impression, into which part of
the tendon of the Tibialis anterior is inserted; in the rest of its extent it
is rough for the attachment of ligaments.
15.2.2.1.3.4.2.2
Lateral Surface
15.2.2.1.3.4.2.2.1
The lateral surface is concave, presenting, along its superior
and posterior borders a narrow L-shaped surface, the vertical limb and
posterior part of the horizontal limb of which articulate with the second
cuneiform, while the anterior part of the horizontal limb articulates with the
second metatarsal bone: the rest of this surface is rough for the attachment of
ligaments and part of the tendon of the Peroneus longus.
15.2.2.1.3.4.2.3
Anterior Surface
15.2.2.1.3.4.2.3.1
The anterior surface, kidney-shaped and much larger than the
posterior, articulates with the first metatarsal bone.
15.2.2.1.3.4.2.4
Posterior Surface
15.2.2.1.3.4.2.4.1
The posterior surface is triangular, concave, and articulates
with the most medial and largest of the three facets on the anterior surface of
the navicular.
15.2.2.1.3.4.2.5
Plantar Surface
15.2.2.1.3.4.2.5.1
The plantar surface is rough, and forms the base of the wedge;
at its back part is a tuberosity for the insertion of part of the tendon of the
Tibialis posterior. It also gives insertion in front to part of the tendon of
the Tibialis anterior.
15.2.2.1.3.4.2.6
Dorsal Surface
15.2.2.1.3.4.2.6.1
The dorsal surface is the narrow end of the wedge, and is directed
upward and lateralward; it is rough for the attachment of ligaments.
15.2.2.1.3.4.3
Articulations
15.2.2.1.3.4.3.1
The first cuneiform articulates with four bones: the
navicular, second cuneiform, and first and second metatarsals.
15.2.2.1.3.5
Second Cuneiform Bone (os cuneiforme secundum; middle
cuneiform)
15.2.2.1.3.5.1
Summary
15.2.2.1.3.5.1.1
The second cuneiform bone, the smallest of the three, is of
very regular wedge-like form, the thin end being directed downward. It is
situated between the other two cuneiforms, and articulates with the navicular
behind, and the second metatarsal in front.
15.2.2.1.3.5.2
Surfaces
15.2.2.1.3.5.2.1
Anterior Surface
15.2.2.1.3.5.2.1.1
The anterior surface, triangular in form, and narrower than
the posterior, articulates with the base of the second metatarsal bone.
15.2.2.1.3.5.2.2
Posterior Surface
15.2.2.1.3.5.2.2.1
The posterior surface, also triangular, articulates with the
intermediate facet on the anterior surface of the navicular.
15.2.2.1.3.5.2.3
Medial Surface
15.2.2.1.3.5.2.3.1
The medial surface carries an L-shaped articular facet,
running along the superior and posterior borders, for articulation with the
first cuneiform, and is rough in the rest of its extent for the attachment of
ligaments.
15.2.2.1.3.5.2.4
Lateral Surface
15.2.2.1.3.5.2.4.1
The lateral surface presents posteriorly a smooth facet for
articulation with the third cuneiform bone.
15.2.2.1.3.5.2.5
Dorsal Surface
15.2.2.1.3.5.2.5.1
The dorsal surface forms the base of the wedge; it is
quadrilateral and rough for the attachment of ligaments.
15.2.2.1.3.5.2.6
Plantar Surface
15.2.2.1.3.5.2.6.1
The plantar surface, sharp and tuberculated, is also rough for
the attachment of ligaments, and for the insertion of a slip from the tendon of
the Tibialis posterior.
15.2.2.1.3.5.3
Articulations
15.2.2.1.3.5.3.1
The second cuneiform articulates with four bones: the
navicular, first and third cuneiforms, and second metatarsal.
15.2.2.1.3.6
Third Cuneiform Bone (os cuneiforme tertium; external
cuneiform)
15.2.2.1.3.6.1
Summary
15.2.2.1.3.6.1.1
The third cuneiform bone, intermediate in size between the two
preceding, is wedge-shaped, the base being uppermost. It occupies the center of
the front row of the tarsal bones, between the second cuneiform medially, the
cuboid laterally, the navicular behind, and the third metatarsal in front.
15.2.2.1.3.6.2
Surfaces
15.2.2.1.3.6.2.1
Anterior Surface
15.2.2.1.3.6.2.1.1
The anterior surface, triangular in form, articulates with the
third metatarsal bone. The posterior surface articulates with the lateral facet
on the anterior surface of the navicular, and is rough below for the attachment
of ligamentous fibers.
15.2.2.1.3.6.2.2
Medial Surface
15.2.2.1.3.6.2.2.1
The medial surface presents an anterior and a posterior
articular facet, separated by a rough depression: the anterior, sometimes
divided, articulates with the lateral side of the base of the second metatarsal
bone; the posterior skirts the posterior border, and articulates with the
second cuneiform; the rough depression gives attachment to an interosseous
ligament.
15.2.2.1.3.6.2.3
Lateral Surface
15.2.2.1.3.6.2.3.1
The lateral surface also presents two articular facets,
separated by a rough non-articular area; the anterior facet, situated at the
superior angle of the bone, is small and semi-oval in shape, and articulates
with the medial side of the base of the fourth metatarsal bone; the posterior
and larger one is triangular or oval, and articulates with the cuboid; the
rough, non-articular area serves for the attachment of an interosseous
ligament. The three facets for articulation with the three metatarsal bones are
continuous with one another; those for articulation with the second cuneiform
and navicular are also continuous, but that for articulation with the cuboid is
usually separate.
15.2.2.1.3.6.2.4
Dorsal Surface
15.2.2.1.3.6.2.4.1
The dorsal surface is of an oblong form, its posterior-lateral
angle being prolonged backward.
15.2.2.1.3.6.2.5
Plantar Surface
15.2.2.1.3.6.2.5.1
The plantar surface is a rounded margin, and serves for the
attachment of part of the tendon of the Tibialis posterior, part of the Flexor
hallucis brevis, and ligaments.
15.2.2.1.3.6.3
Articulations
15.2.2.1.3.6.3.1
The third cuneiform articulates with six bones: the navicular,
second cuneiform, cuboid, and second, third, and fourth metatarsals.
15.2.2.2
Metatarsus
(5)
15.2.2.2.1
Summary
15.2.2.2.1.1
The metatarsus consists of five metatarsal bones numbered 1-5
from the medial to lateral position.
15.2.2.2.1.2
Like the metacarpals of the palm of the hand, each metatarsal
consists of a proximal base, a shaft, with a distal head.
15.2.2.2.1.3
The metatarsals articulate proximally with the first, second
and third cuneiform bones and with the cuboid.
15.2.2.2.1.4
Distally, they articulate with the proximal row of phalanges.
15.2.2.2.1.5
The first metatarsal is thicker than the others because it
bears more weight.
15.2.2.2.2
Detailed
Description
15.2.2.2.2.1
Introduction
15.2.2.2.2.1.1
The metatarsus consists of five bones which are numbered from
the medial side (ossa metatarsalia I.-V.); each presents for examination a body
and two extremities.
15.2.2.2.2.2
Common Characteristics of the Metatarsal Bones
15.2.2.2.2.2.1
The body is prismoid in form, tapers
gradually from the tarsal to the phalangeal extremity, and is curved
longitudinally, so as to be concave below, slightly convex above.
15.2.2.2.2.2.2
Base or Posterior Extremity
15.2.2.2.2.2.2.1
The base or posterior extremity is wedge-shaped, articulating
proximally with the tarsal bones, and by its sides with the contiguous
metatarsal bones: its dorsal and plantar surfaces are rough for the attachment
of ligaments.
15.2.2.2.2.2.3
Head or Anterior Extremity
15.2.2.2.2.2.3.1
The head or anterior extremity presents a convex articular
surface, oblong from above downward, and extending farther backward below than
above. Its sides are flattened, and on each is a depression, surmounted by a
tubercle, for ligamentous attachment. Its plantar surface is grooved
anterior-posteriorly for the passage of the Flexor tendons, and marked on either
side by an articular eminence continuous with the terminal articular surface.
15.2.2.2.2.2.4
Characteristics of the Individual Metatarsal Bones
15.2.2.2.2.2.4.1
The First Metatarsal Bone (os metatarsale I; metatarsal bone
of the great toe)
15.2.2.2.2.2.4.1.1
The first metatarsal bone is remarkable for its great
thickness, and is the shortest of the metatarsal bones. The body is strong, and
of well-marked prismoid form. The base presents, as a rule, no articular facets
on its sides, but occasionally on the lateral side there is an oval facet, by
which it articulates with the second metatarsal. Its proximal articular surface
is of large size and kidney-shaped; its circumference is grooved, for the
tarsometatarsal ligaments, and medially gives insertion to part of the tendon
of the Tibialis anterior; its plantar angle presents a rough oval prominence
for the insertion of the tendon of the Peronćus longus. The head is large; on
its plantar surface are two grooved facets, on which glide sesamoid bones; the
facets are separated by a smooth elevation.
15.2.2.2.2.2.4.2
The Second Metatarsal Bone (os metatarsale II)
15.2.2.2.2.2.4.2.1
The second metatarsal bone is the longest of the metatarsal
bones, being prolonged backward into the recess formed by the three cuneiform
bones. Its base is broad above, narrow and rough below. It presents four
articular surfaces: one behind, of a triangular form, for articulation with the
second cuneiform; one at the upper part of its medial surface, for articulation
with the first cuneiform; and two on its lateral surface, an upper and lower,
separated by a rough non-articular interval. Each of these lateral articular
surfaces is divided into two by a vertical ridge; the two anterior facets
articulate with the third metatarsal; the two posterior (sometimes continuous)
with the third cuneiform. A fifth facet is occasionally present for
articulation with the first metatarsal; it is oval in shape, and is situated on
the medial side of the body near the base.
15.2.2.2.2.2.4.3
The Third Metatarsal Bone (os metatarsale III)
15.2.2.2.2.2.4.3.1
The third metatarsal bone articulates proximally, by means of
a triangular smooth surface, with the third cuneiform; medially, by two facets,
with the second metatarsal; and laterally, by a single facet, with the fourth
metatarsal. This last facet is situated at the dorsal angle of the base.
15.2.2.2.2.2.4.4
The Fourth Metatarsal Bone (os metatarsale IV)
15.2.2.2.2.2.4.4.1
The fourth metatarsal bone is smaller in size than the
preceding; its base presents an oblique quadrilateral surface for articulation
with the cuboid; a smooth facet on the medial side, divided by a ridge into an
anterior portion for articulation with the third metatarsal, and a posterior
portion for articulation with the third cuneiform; on the lateral side a single
facet, for articulation with the fifth metatarsal.
15.2.2.2.2.2.4.5
The Fifth Metatarsal Bone (os metatarsale V)
15.2.2.2.2.2.4.5.1
The fifth metatarsal bone is recognized by a rough eminence,
the tuberosity, on the lateral side of its base. The base articulates behind,
by a triangular surface cut obliquely in a transverse direction, with the
cuboid; and medially, with the fourth metatarsal. On the medial part of its
dorsal surface is inserted the tendon of the Peroneus Tertius and on the dorsal
surface of the tuberosity that of the Peroneus brevis. A strong band of the
plantar aponeurosis connects the projecting part of the tuberosity with the
lateral process of the tuberosity of the calcaneus. The plantar surface of the
base is grooved for the tendon of the Abductor digiti quinti, and gives origin
to the Flexor digiti quinti brevis.
15.2.2.2.2.3
Articulations
15.2.2.2.2.3.1
The base of each metatarsal bone articulates with one or more
of the tarsal bones, and the head with one of the first row of phalanges. The
first metatarsal articulates with the first cuneiform, the second with all
three cuneiforms, the third with the third cuneiform, the fourth with the third
cuneiform and the cuboid, and the fifth with the cuboid.
15.2.2.3
Phalanges
(14)
15.2.2.3.1
Summary
15.2.2.3.1.1
The phalanges of the foot resemble those of the hand both in
number and arrangement. Each also consists of a proximal base, a middle shaft,
and a distal head.
15.2.2.3.1.2
The Hallux (great or big toe), has two large, heavy phalanges
called proximal and distal phalanges.
15.2.2.3.1.3
The other four toes each have three phalanges, proximal,
middle, and distal.
15.2.2.3.2
Detailed
Description
15.2.2.3.2.1
Introduction
15.2.2.3.2.1.1
The phalanges of the foot correspond, in number and general
arrangement, with those of the hand; there are two in the great toe, and three
in each of the other toes. They differ from them, however, in their size, the
bodies being much reduced in length, and, especially in the first row,
laterally compressed.
15.2.2.3.2.2
First Row
15.2.2.3.2.2.1
The body of each is compressed from side to side, convex
above, concave below. The base is concave; and the head presents a trochlear
surface for articulation with the second phalanx.
15.2.2.3.2.3
Second Row
15.2.2.3.2.3.1
The phalanges of the second row are remarkably small and
short, but rather broader than those of the first row.
15.2.2.3.2.4
The ungual phalanges
15.2.2.3.2.4.1
The ungual phalanges, in form, resemble those of the fingers;
but they are smaller and are flattened from above downward; each presents a
broad base for articulation with the corresponding bone of the second row, and
an expanded distal extremity for the support of the nail and end of the toe.
15.2.2.3.2.5
Articulations
15.2.2.3.2.5.1
In the second, third, fourth, and fifth toes the phalanges of the
first row articulate behind with the metatarsal bones, and in front with the
second phalanges, which in their turn articulate with the first and third: the
ungual phalanges articulate with the second.
15.2.2.4
Total
Bones of Foot=26
15.2.3
Arches
of the Foot
15.2.3.1
The
bones of the foot are arranged in two arches. These arches enable the foot to
support the weight of the body, provide an ideal distribution of body weight
over the hard and soft tissues of the foot, and provide leverage while walking.
15.2.3.2
While
these medial and lateral arches may be readily demonstrated as the component
antero-posterior arches of the foot, yet the fundamental longitudinal arch is
contributed to by both, and consists of the calcaneus, cuboid, third cuneiform,
and third metatarsal: all the other bones of the foot may be removed without
destroying this arch.
15.2.3.3
The
arches are not rigid. They yield as weight is applied and spring back when the
weight is lifted, thus helping to absorb shocks.
15.2.3.4
Longitudinal
Arch
15.2.3.4.1
The
longitudinal arch has two parts. Both consist of tarsal and metatarsal bones
arranged to form an arch from the anterior to the posterior part of the foot.
15.2.3.4.1.1
Medial Longitudinal Arch
15.2.3.4.1.1.1
Originates at the calcaneus, rises to the talus and descends
through the Navicular, the three cuneiforms, and the heads of the three medial
metatarsals.
15.2.3.4.1.1.2
The talus is the keystone of the arch.
15.2.3.4.1.1.3
Its summit is at the superior articular surface of the talus,
and its two extremities or piers, on which it rests in standing, are the
tuberosity on the plantar surface of the calcaneus posteriorly and the heads of
the first, second, and third metatarsal bones anteriorly.
15.2.3.4.1.1.4
The chief characteristic of this arch is its elasticity, due
to its height and to the number of small joints between its component parts.
15.2.3.4.1.1.5
Its weakest part, i. e., the part most liable to yield from
overpressure, is the joint between the talus and Navicular, but this portion is
braced by the plantar calcaneonavicular ligament, which is elastic and is thus
able to quickly restore the arch to its pristine condition when the disturbing
force is removed.
15.2.3.4.1.1.6
The ligament is strengthened medially by blending with the
deltoid ligament of the ankle-joint, and is supported inferiorly by the tendon
of the Tibialis posterior, which is spread out in a fan shaped insertion and
prevents undue tension of the ligament or such an amount of stretching as would
permanently elongate it.
15.2.3.4.1.1.7
The arch is further supported by the plantar aponeurosis, by
the small muscles in the sole of the foot, by the tendons of the Tibialis
anterior and posterior and Peronćus longus, and by the ligaments of all the
articulations involved.
15.2.3.4.1.2
Lateral Longitudinal Arch
15.2.3.4.1.2.1
Begins at the calcaneus, rises at the cuboid and descends to
the heads of the two lateral metatarsals.
15.2.3.4.1.2.2
The cuboid is the keystone of this arch.
15.2.3.4.1.2.3
The calcaneocuboid, possesses a special mechanism for locking,
and allows only a limited movement
15.2.3.4.1.2.4
The most marked features of this arch are its solidity and its
slight elevation; two strong ligaments, the long plantar and the plantar
calcaneocuboid, together with the Extensor tendons and the short muscles of the
little toe, preserve its integrity.
15.2.3.4.2
Transverse
Arch
15.2.3.4.2.1
The Navicular, three cuneiforms, cuboid, and the bases of the
five metatarsals form the transverse arch.
15.2.3.4.2.2
At the posterior part of the metatarsus and the anterior part
of the tarsus the arches are complete, but in the middle of the tarsus they
present more the characters of half-domes the concavities of which are directed
downward and medial ward, so that when the medial borders of the feet are
placed in apposition a complete tarsal dome is formed.
15.2.3.4.2.3
The transverse arches are strengthened by the interosseous,
plantar, and dorsal ligaments, by the short muscles of the first and fifth toes
(especially the transverse head of the Adductor hallucis), and by the Peroneus
longus, whose tendon stretches across between the piers of the arches.
15.2.4
15.3 Arthrology
15.3.1
Tibiofibular Joint
15.3.1.1 Summary
15.3.1.1.1
The superior and inferior tibiofibular joints are separate
from the ankle joint but must provide accessory movements for full ankle function.
15.3.1.1.2
The superior Tibiofibular is formed between the proximal ends
of the tibia and fibula when the fibular head joins with a facet on the
Posterolateral aspect of the rim of the tibial condyle forming a plane synovial
joint.
15.3.1.1.3
The inferior Tibiofibular joint is formed between the distal
ends of the tibia and fibula forming a Syndesmosis with fibroadipose tissue,
and bound together by the Crural Tibiofibular interosseous ligament and the
anterior and posterior Tibiofibular ligaments.
15.3.1.1.4
With Dorsiflexion, plantarflexion, inversion, and Eversion
there are slight accessory movements of the fibula.
15.3.1.2 Introduction
15.3.1.2.1
Anatomically, the superior and inferior tibiofibular joints
are separate from the ankle but provide accessory motions, which allow greater
movement at the ankle, fusion or immobility in these joint may impair ankle
function.
15.3.1.3 Superior Tibiofibular Joint
15.3.1.3.1
Definition
15.3.1.3.1.1
This joint is formed between the proximal ends of the tibia
and fibula
15.3.1.3.1.2
The fibular head joins with a facet on the Posterolateral
aspect of the rim of the tibial condyle; the facet faces posteriorly,
inferiorly, and laterally.
15.3.1.3.2
Type of Joint
15.3.1.3.2.1
Plane synovial joint
15.3.1.4 Inferior Tibiofibular Joint
15.3.1.4.1
Discussion
15.3.1.4.1.1
The inferior
tibiofibular joint is a Syndesmosis and lacks articular cartilage and synovium.
The distal fibula is situated in the fibular notch of the lateral aspect of
the distal tibia and is bound to it by several ligaments (Figs. 15-10 and
15-11). The anterior and posterior tibiofibular ligaments pass in front of and
behind the Syndesmosis. They are both directed downward and inward to check
separation of the two bones. The inferior transverse ligament is a thickened
band of fibers that is closely related to the posterior tibiofibular ligament.
It passes from the posterior margin of the inferior tibial articular surface
downward and laterally to the malleolar fossa of the fibula. This ligament is
lined inferiorly with articular cartilage where it contacts the posterolateral talar
articular surface during extreme plantar flexion. The interosseous ligament is
a continuation of the interosseous membrane of the tibia and
fibula. It extends between the adjacent surfaces of the bones at the
Syndesmosis. The tibia and fibula are separated at the syndesmosis by a fat
pad.
15.3.1.4.1.2
The ligaments of the inferior tibiofibular articulation are
oriented to prevent widening of the mortise. They are also important in
preventing posterior displacement of the fibula at the syndesmosis, which tends
to occur when the leg is forcibly internally rotated on the tarsus. It should be realized
that complete sectioning of the inferior tibiofibular ligaments alone
allows only a minimal increase in. the intermalleolar space. This is because
the two bones are indirectly held together by their mutual connections to the
talus, by way of the medial and lateral ligaments of the ankle. Significant
diastasis, then, is usually accompanied by rupture of one or more of
the Talocrural
ligaments, usually the deltoid ligament.
15.3.1.4.1.3
Figure 15-10-11
15.3.1.4.1.3.1
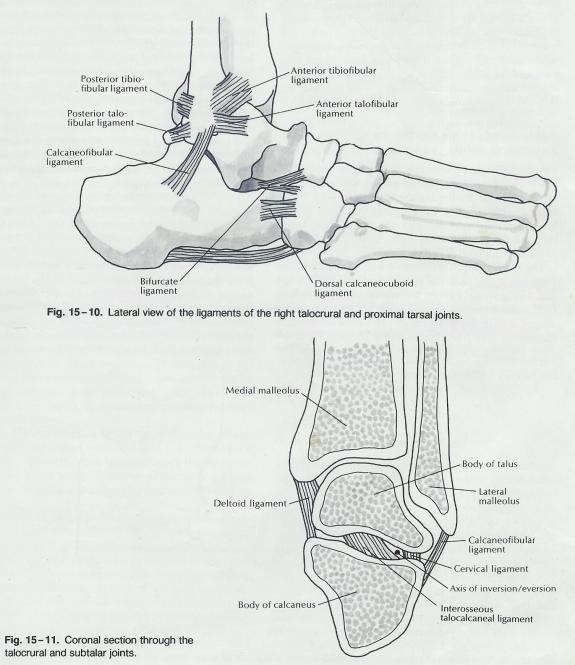
15.3.1.4.2
Definition
15.3.1.4.2.1
This joint is formed between the distal ends of the tibia and
fibula
15.3.1.4.3
Type of Joint
15.3.1.4.3.1
Syndesmosis with fibroadipose tissue between the two bony
surfaces
15.3.1.4.4
Ligaments
15.3.1.4.4.1
Crural Tibiofibular Interosseous Ligament
15.3.1.4.4.2
Anterior and Posterior Tibiofibular ligaments
15.3.1.5 Movements
15.3.1.5.1
With Dorsiflexion and plantarflexion of the ankle, there are
slight accessory movements of the fibula
15.3.1.5.1.1
Plantar Flexion & Dorsiflexion
15.3.1.5.1.2
As the ankle plantarflexes, the lateral Malleolus (fibula) rotates
medially and is pulled inferiorly, and the two malleoli approximate.
15.3.1.5.1.3
At the superior joint, the fibula slides inferiorly
15.3.1.5.1.4
The opposite occurs with Dorsiflexion
15.3.1.5.1.5
Supination and Inversion
15.3.1.5.1.6
As the ankle supinates and inverts, the head of the fibula
slides distally and posteriorly (external rotation)
15.3.1.5.1.7
Pronation and Eversion
15.3.1.5.1.8
The head of the fibula slides proximally and anteriorly
(internal rotation)
15.3.1.6 Nerve Supply
15.3.1.6.1
Proximal (Superior) Tibiofibular Joint
15.3.1.6.2
Distal (Inferior) Tibiofibular Joint
15.3.2
Talocrural (Ankle) Joint
(ta’’lo-krōōr’al)
15.3.2.1 Summary
15.3.2.1.1
This Synovial Hinge (Ginglymus) Type joint is formed by the
congruous union of the distal end of the tibia and its medial Malleolus and the
Lateral Malleolus of the fibula both forming the concave articulating surface
of the mortice with the convex talar surface.
15.3.2.1.2
The fibrous capsule is lined by a synovial membrane throughout
its entirety and well supported by the deltoid ligament medially and the
lateral collateral ligaments (Anterior Talofibular, Posterior Talofibular, and
Calcaneofibular).
15.3.2.1.3
Since the axis of movement, (20ş Dorsiflexion and 45ş
plantarflexion) is near the tip of both malleoli these ligaments are not pulled
tight during normal movement at the Talocrural joint.
15.3.2.2 Illustration
15.3.2.3 Introduction
15.3.2.3.1
The ankle joint is formed by the superior portion of the body
of the talus fitting within the mortise, or cavity, formed by the combined
distal ends of the tibia and fibula.
15.3.2.3.2
The medial, superior, and lateral articular surfaces of the
talus are continuous, as are those of the medial Malleolus, the distal end of
the tibia, and the lateral Malleolus.
15.3.2.3.3
The fibrous capsule attaches at the margins of the articular
surfaces of the talus below and to the tibia and fibula above, except
anteriorly, where a portion of the dorsal aspect of the neck of the talus is
enclosed within the joint cavity.
15.3.2.3.4
The capsule extends somewhat superiorly between the distal
ends of the tibia and fibula, to just below the Syndesmosis.
15.3.2.3.5
The fibrous capsule is lined by a synovial membrane throughout
its entirety and well supported by ligaments, especially medially and
laterally.
15.3.2.3.6
The concave articulating surface is the mortice, which is made
up of the distal tibia and the tibial and fibular malleoli. The fibular
Malleolus extends further distally than the tibial Malleolus. The combined
surfaces are congruent with the body of the talus.
15.3.2.3.7
Integrity of the mortice is provided by the tibiofibular
joints and their associated ligaments
15.3.2.3.8
The convex articulating surface is the body of the talus.
15.3.2.3.9
The medial ligaments are collectively referred to as the deltoid ligament (Fig. 15-12). The
anterior portion of the deltoid ligament consists of the Tibionavicular
ligament, superficially, and the deeper anterior Tibiotalar fibers. The
Tibionavicular ligament blends with the plantar calcaneonavicular (spring)
ligament inferiorly. The middle fibers of the deltoid ligament constitute the
tibiocalcaneal ligament, with some Tibiotalar fibers deep to it. The posterior
Tibiotalar ligament forms the posterior portion of the deltoid ligament. The
deltoid ligament as a whole attaches proximally to the medial aspect of the medial
malleolus and fans out to achieve the distal attachments described above. In
this way, it is somewhat triangular, with the apex at its proximal attachment.
15.3.2.3.10
The lateral ligaments, unlike those of the medial side, are
separate bands of fibers diverging from their proximal attachment at the distal end of the
fibula (see Fig. 15 - 10). The anterior talofibular ligament the
most frequently injured ligament about the ankle-passes medially, forward and
downward, from the anterior aspect of the fibula to the lateral aspect of the
neck of the talus. The calcaneofibular ligament runs from the tip of the
lateral malleolus downward and backward to a small prominence on the upper
lateral surface of the calcaneus. It is longer and narrower than the anterior
and posterior talofibular ligaments. The posterior talofibular ligament passes
from the malleolar fossa medially and slightly downward and backward to the
lateral tubercle of the posterior aspect of the talus.
15.3.2.3.11
It should be noted that the proximal attachments of
both the medial and lateral ligaments of the ankle are near the axis of
movement for dorsiflexion and plantar flexion. For this reason, these ligaments
are not pulled tight to any significant extent during normal movement at the
Talocrural joint.[6]
Also, the calcaneofibular ligament, which crosses both the talocrural and the
talocalcaneal joints, runs parallel to, and inserts close to, the axis of
movement at the subtalar joint. It, then, plays little or no role in
restricting inversion at the subtalar joint. This is true in all positions of
dorsiflexion and plantar flexion, since it maintains a parallel orientation to the
subtalar axis throughout the range.
15.3.2.3.12
The ligaments about the talocrural joint primarily function to
restrict titling and rotation of the talus within the mortise and to restrict
forward or backward displacement of the leg on the tarsus. The main exception
to this is the tibiocalcaneal portion of the deltoid ligament, which is so
oriented as to help check eversion at the subtalar joint as well as an
"eversion tilt" of the talus in the mortise.
15.3.2.3.13
In the neutral position, the anterior talofibular ligament
can check posterior movement of the leg on the tarsus and external rotation of
the leg on the tarsus because it is directed forward and medially. With the
foot in plantar flexion, the anterior talofibular ligament becomes more
vertically oriented and is in a position to check inversion of the talus in.
the mortise. This ligament is the most commonly injured of the ligaments of the
ankle, the mechanism of injury usually being a combined plantar flexion inversion
strain.
15.3.2.3.14
The calcaneofibular ligament is directed downward and
backward when the foot is in the neutral position. When the foot is
dorsiflexed, the ligament becomes more vertically oriented and is in a better
position to check inversion of the tarsus with respect to the leg.
15.3.2.3.15
The posterior talofibular ligaments oriented so as to check
internal rotation of the leg on the tarsus and forward displacement of the leg
on the tarsus.
15.3.2.3.16
The deltoid ligament, considered as a whole, contributes to
restriction of eversion, internal rotation, and external rotation, as well as
forward and backward displacement of the tarsus. However, sectioning of the
deltoid ligament alone apparently results primarily in instability into
eversion of the tarsus on the tibia, the other motions being checked by other
ligaments, as described previously.
15.3.2.3.17
Figure 15-12
15.3.2.3.17.1
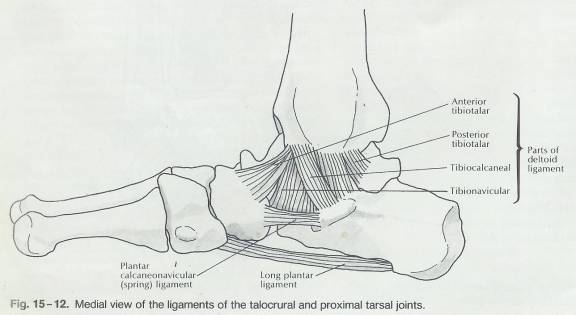
15.3.2.3.18
Figure 15-13
15.3.2.3.18.1
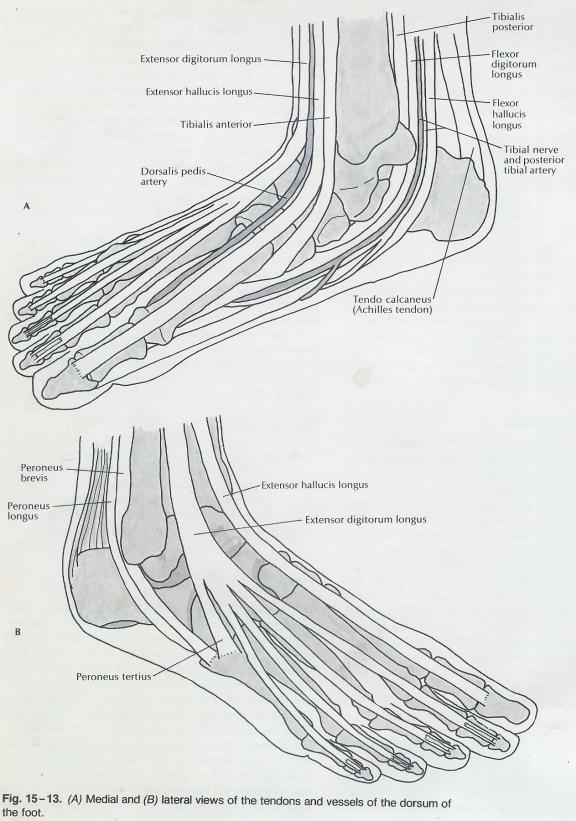
15.3.2.4 Definition
15.3.2.4.1
This joint is formed between the Talus and two bones
15.3.2.4.1.1
Distal end of the tibia and its medial Malleolus
15.3.2.4.1.2
Lateral Malleolus of the fibula
15.3.2.5 Type of Joint
15.3.2.5.1
Synovial
15.3.2.5.2
Hinge (Ginglymus) Type
15.3.2.6 Movements
15.3.2.6.1
Axis of Movement
15.3.2.6.1.1
Extends obliquely from the Posterolateral aspect of the
fibular Malleolus to the Anteromedial aspect of the tibial Malleolus.
15.3.2.6.2
Degree of Movement
15.3.2.6.2.1
With the knee flexed, the ankle joint can be dorsiflexed about
20ş.
15.3.2.6.2.2
If the knee is extended, the Gastrocnemius will limit the
range of motion to about 10ş.
15.3.2.6.2.3
The range of motion in plantar flexion is approximately 45ş
15.3.2.6.3
Dorsiflexion 20ş
15.3.2.6.3.1
Body of the talus slides in a posterior direction
15.3.2.6.4
Plantar Flexion 45ş
15.3.2.6.4.1
Body of the talus slides in a anterior direction
15.3.2.7 Ligaments
15.3.2.7.1
Introduction
15.3.2.7.1.1
The proximal attachments of both the medial and lateral
ligaments of the ankle are near the axis of movement for Dorsiflexion and
plantar flexion.
15.3.2.7.1.2
As a result, these ligaments are not pulled tight to any
significant extent during normal movement at the Talocrural joint.
15.3.2.7.1.3
The ligaments about the Talocrural joint primarily function to
restrict tilting and rotation of the talus within the mortise and to restrict
forward or backward displacement of the leg on the tarsus.
15.3.2.7.1.4
The main exception to this is the Tibiocalcaneal portion of
the deltoid ligament, which is so oriented as to help check eversion at the Subtalar
joint as well as an “eversion tilt” of the talus in the mortise.
15.3.2.7.2
Medial (deltoid) Ligaments
15.3.2.7.2.1
Introduction
15.3.2.7.2.2
The deltoid ligament as a whole attaches proximally to the
medial aspect of the medial Malleolus and fans out to achieve the distal
attachments described above.
15.3.2.7.2.3
It is somewhat triangular, with the apex at its proximal
attachment
15.3.2.7.2.4
The deltoid ligament, considered as a whole, contributes to
restriction of eversion, internal rotation, and external rotation, as well as
forward and backward displacement of the tarsus. However, sectioning of the
deltoid ligament alone apparently results primarily in stability into eversion
of the tarsus on the tibia, the other motions being checked by other ligaments,
as described.
15.3.2.7.2.5
Anterior Tibiotalar
15.3.2.7.2.6
Posterior Tibiotalar
15.3.2.7.2.7
Tibiocalcaneal
15.3.2.7.2.8
Tibionavicular
15.3.2.7.2.9
The Tibionavicular ligament blends with the plantar
calcaneonavicular (spring) ligament inferiorly
15.3.2.7.3
Lateral Collateral Ligaments
15.3.2.7.3.1
Introduction
15.3.2.7.3.1.1
The lateral ligaments, unlike those of the medial side, are
separate bands of fibers diverging from their proximal attachment at the distal
end of the fibula.
15.3.2.7.3.2
Anterior Talofibular
15.3.2.7.3.2.1
The anterior Talofibular ligament is the most frequently
injured ligament in ankle sprains
15.3.2.7.3.2.2
It passes medially, forward and downward from the anterior aspect
of the fibula to the lateral aspect of the neck of the talus.
15.3.2.7.3.2.3
In the neutral position, the anterior Talofibular ligament can
check posterior movement of the leg on the tarsus and external rotation of the
leg on the tarsus because it is directed forward and medially. With the foot in
plantar flexion, the anterior Talofibular ligament becomes more vertically
oriented and is in a position to check inversion of the talus in the mortise.
This ligament is the most commonly injured of the ligaments of the ankle, the
mechanism of injury usually being a combined plantar flexion-inversion strain.
15.3.2.7.3.3
Posterior Talofibular
15.3.2.7.3.3.1
Passes from the Malleolar fossa medially and slightly downward
and backward to the lateral tubercle of the posterior aspect of the talus.
15.3.2.7.3.3.2
The posterior Talofibular ligaments is oriented so as to check
internal rotation of the leg on the tarsus and forward displacement of the leg
on the tarsus.
15.3.2.7.3.4
Calcaneofibular
15.3.2.7.3.4.1
Runs from the tip of the lateral Malleolus downward and
backward to a small prominence on the upper lateral surface of the calcaneus.
15.3.2.7.3.4.2
It is longer and narrower than the anterior and posterior
Talofibular ligaments.
15.3.2.7.3.4.3
The Calcaneofibular ligament is directed downward and backward
when the foot is in the neutral position. When the foot is dorsiflexed, the
ligament becomes more vertically oriented and is in a better position to check
inversion of the tarsus with respect to the leg.
15.3.2.7.3.4.4
The Calcaneofibular ligament, which crosses both the
Talocrural and the talocalcaneal joint, runs parallel to, and inserts close to,
the axis of movement at the Subtalar joint. The Calcaneofibular ligament plays
little or no role in restricting inversion at the Subtalar joint. This is true
in all positions of Dorsiflexion and plantar flexion, since it maintains a
parallel orientation to the Subtalar axis throughout the range.
15.3.2.8 Nerve Supply
15.3.3
Subtalar/Talocalcaneal
(ta’’lo-kal-ka-ne-al)
15.3.3.1 Summary
15.3.3.1.1
This synovial modified plane or gliding joint is uniaxial and
is formed by the union of the talus and calcaneus at two separate articular
capsules (Posterior & Anterior) separated by the tarsal canal which divides
the two joint cavities.
15.3.3.1.2
The Subtalar joint allows the movements of inversion and
eversion. This joint is supported by several external ligaments (Medial and
Lateral Collateral Ligaments (Talocrural), Extensor Retinaculum, Posterior and
Lateral Talocalcaneal) and internal ligaments (Interosseous Talocalcaneal
(Tarsal Canal) & Cervical Talocalcaneal Ligament)
15.3.3.2 Illustration
15.3.3.3 Introduction
15.3.3.3.1
The talus also articulates with the Navicular, and the
Talonavicular joint is involved in the movements ascribed to the Subtalar
joint.
15.3.3.3.2
Functionally, the Subtalar joint includes the articulation
between the posterior facet of the talus and the opposing articular surface of
the calcaneus, as well as the articulation between the anterior and medial
facets of the two bones.
15.3.3.3.3
These articulations move in conjunction with one another.
Anatomically, the anterior and medial articulations are actually part of the
talocalcaneonavicular joint; they are enclosed within a joint capsule separate
from that of the posterior talocalcaneal articulation.
15.3.3.3.4
Functionally, the subtalar joint includes the articulation
between the posterior facet of the talus and the opposing articular surface of
the calcaneus, as well as the articulation between the anterior and medial facets of the two bones. These articulations
move in conjunction with one another. Anatomically, the anterior and
medial articulations are actually part of the talocalcaneonavicular joint; they
are enclosed within a joint capsule separate from that of the posterior
talocalcaneal articulation.
15.3.3.3.5
. The joint capsules of the posterior portion
of the talocalcaneal joint and the talocalcaneonavicular portion of the
subtalar joint are separated by the ligament of the tarsal canal. This
ligament runs from the underside of the talus, at the sulcus tali, downward and
laterally to the dorsum of the calcaneus, at the sulcus calcanei. Since it is
situated medially to the axis of motion of inversion-eversion at the subtalar
joint, it checks eversion.[7] This
ligament is often referred to as the interosseous talocalcaneal ligament (see Fig. 15-11).
15.3.3.3.6
More laterally, in the sinus tarsi, is the cervical
talocalcaneal ligament. It passes from the inferolateral aspect of the talar
neck downward and laterally to the dorsum of the calcaneus. It occupies the
anterior part of the sinus tarsi. Since the cervical ligament lies lateral to
the subtalar joint axis, it restricts inversion of the calcaneus on the talus.[8]
15.3.3.3.7
Also, within the lateral aspect of the sinus tarsi, bands from
the inferior aspect of the extensor retinaculum pass downward, as well as medially,
to the calcaneus. These bands are considered part df the talocalcaneal ligament
complex. They help check inversion at the subtalar joint.
15.3.3.4 Definition
15.3.3.4.1
This joint is formed between the Talus and calcaneus.
15.3.3.5 Type of Joint
15.3.3.5.1
Synovial
15.3.3.5.2
Modified plane or gliding joint
15.3.3.6 Movements
15.3.3.6.1
Supination and pronation are movements permitted by the
Subtalar and talocalcaneonavicular joint.
15.3.3.6.2
Uniaxial
15.3.3.6.3
Inversion (Kisner[9])
15.3.3.6.3.1
Turning inward of the calcaneus and is combined with
adduction, supination, and plantarflexion of the Talonavicular joint
15.3.3.6.4
Eversion (Kisner[10])
15.3.3.6.4.1
Turning outward of the calcaneus and is combined with
abduction, pronation, and Dorsiflexion of the Talonavicular joint
15.3.3.6.5
Supination (Kendall[11])
15.3.3.6.5.1
Rotation of the foot in which the sole of the foot moves in a
medial direction
15.3.3.6.6
Pronation (Kendall[12])
15.3.3.6.6.1
Rotation in which the sole of the foot moves in a lateral
direction
15.3.3.7 Ligaments
15.3.3.7.1
Medial and Lateral Collateral Ligaments (Talocrural)
15.3.3.7.2
Extensor Retinaculum
15.3.3.7.2.1
Also, within the lateral aspect of the sinus tarsi bands from
the inferior aspect of the extensor retinaculum pass downward, as well as
medially, to the calcaneus. These bands are considered part of the
talocalcaneal ligament complex. They help check inversion at the Subtalar
joint.
15.3.3.7.3
Interosseous Talocalcaneal (Tarsal Canal)
15.3.3.7.3.1
The ligament of the tarsal canal separates the joint capsules
of the posterior portion of the talocalcaneal joint and the
talocalcaneonavicular portion of the Subtalar joint. This ligament runs from
the underside of the talus, at the Sulcus tali, downward and laterally to the
dorsum of the calcaneus at the Sulcus calcanei. Since it is situated medially
to the axis of motion of inversion-eversion at the Subtalar joint, it checks
eversion. This ligament is often referred to as the interosseous talocalcaneal
ligament.
15.3.3.7.4
Cervical Talocalcaneal Ligament
15.3.3.7.4.1
More laterally, in the sinus tarsi, is the cervical
talocalcaneal ligament. It passes from the inferolateral aspect of the talar
neck downward and laterally to the dorsum of the calcaneus. It occupies the
anterior part of the sinus tarsi. Since the cervical ligament lies lateral to
the Subtalar joint axis, it restricts inversion of the calcaneus on the talus.
15.3.3.7.5
Posterior and Lateral Talocalcaneal
15.3.3.8 Articulations
15.3.3.8.1
There are three articulations between the talus and calcaneus;
the posterior is separated from the anterior and middle by the tarsal canal.
The canal divides the Subtalar joint into two joint cavities.
15.3.3.8.2
With physiologic motions of the Subtalar joint, the convex
posterior portion of the calcaneus slides opposite to the motion; the concave
anterior and middle facets on the calcaneus slide in the same direction.
15.3.3.8.2.1
Inversion
15.3.3.8.2.1.1
Posterior Articulation Slides Lateral
15.3.3.8.2.2
Eversion
15.3.3.8.2.2.1
Posterior Articulation Slides Medial
15.3.3.8.3
Posterior Articulation
15.3.3.8.3.1
Has its own capsule; the facet on the bottom of the talus is
concave, while the opposing facet on the calcaneus is convex.
15.3.3.8.4
Anterior Articulations
15.3.3.8.4.1
Enclosed in the same capsule as the Talonavicular
articulation, forming the talocalcaneonavicular joint. Functionally, these
articulations work together. The facets of the anterior and middle
articulations on the talus are convex, while the opposing facets on the
calcaneus are concave.
15.3.3.9 Nerve Supply
15.3.4
Talocalcaneonavicular (TCN)
15.3.4.1 Summary
15.3.4.1.1
This Synovial, compound ball & socket joint with the
Navicular forming the socket and the talus forming the ball. The talus
articulates with the calcaneus at anterior and medial facets. All of the above
articulations are enclosed in the same fibrous capsule. The joint is supported
by the calcaneonavicular (Spring) ligament, Bifurcate Ligament
(Calcaneonavicular Portion), Deltoid Ligament (Tibionavicular Portion), and
Dorsal Talonavicular ligament. The joint assists in inversion and eversion.
15.3.4.2 Illustration
15.3.4.3 Introduction
15.3.4.3.1
The TCN joint includes the articulation between the anterior
and medial facets of the talus and calcaneus (Subtalar/Talocalcaneal Joint),
the articulations between the inferior aspect of the head of the talus and the
subjacent spring ligament, and the articulation between the anterior aspect of
the head of the talus and the posterior articular surface of the Navicular.
15.3.4.3.2
The Talonavicular portion of this joint constitutes the medial
half of the transverse tarsal joint.
15.3.4.3.3
The talocalcaneonavicular joint is enclosed by a joint
capsule, the posterior aspect of which transverses the tarsal canal, forming
the anterior wall of the canal. The capsule is reinforced by the spring
ligament inferiorly, the calcaneonavicular portion to the bifurcate ligament
laterally, and the Tibionavicular portion of the deltoid ligament medially.
15.3.4.3.4
The talocalcaneonavicular joint includes the articulation
between the anterior and medial facets of the talus and calcaneus (described
previously as part of the subtalar joint), the articulations between the
inferior aspect of the head of the talus and the subjacent spring ligament, and
the articulation between the anterior aspect of the head of the talus and the
posterior articular surface of the navicular. The combined talonavicular and
talo-spring ligament portion of this joint is essentially a compound ball-and socket joint; the
head of the talus is the ball, while the superior surface of t4e spring
ligament and the posterior surface of the navicular form: the socket. It should be noted that the
superior surface of the spring ligament is lined with articular cartilage. The
talonavicular portion of this joint constitutes the medial half of the
transverse tarsal joint..
15.3.4.3.5
The talocalcaneonavicular joint is enclosed by a joint
capsule, the posterior aspect of which traverses the tarsal canal, forming the
anterior wall of the canal.. The capsule, is reinforced by the spring ligament
inferiorly, the calcaneonavicular portion of the bifurcate ligament laterally,
and the tibionavicular portion of the deltoid ligament medially (see Figs.
15-10 and 15-12).
15.3.4.3.6
The spring ligament passes from the anterior and medial
margins of the sustentaculum tali forward to the inferior and inferomedial
aspect of the navicular.
15.3.4.3.7
. As mentioned, its superior surface articulates with the
underside of the head of the talus. This ligament maintains apposition of the
medial aspects of the forefoot and hindfoot and in so doing helps to maintain
the normal arched configuration of the foot. Laxity of the ligament allows. a
medial separation between the calcaneus and forefoot, with the forefoot
assuming an abducted position with respect to the hindfoot. At the same time,
the foot is allowed to "untwist," which effectively lowers the normal
arch of the foot, and the talar head is allowed to move medially and inferiorly.
Further discussion of the twisted configuration and arching of the foot is included
in the section on biomechanics.
15.3.4.4 Definition
15.3.4.4.1
This joint is formed between the anterior capsule of the
Subtalar/Talocalcaneal Joint and the Navicular.
15.3.4.5 Type of Joint
15.3.4.5.1
Synovial
15.3.4.5.2
Compound Ball & Socket
15.3.4.5.2.1
The combined Talonavicular and talo-spring ligament portion of
this joint is essentially a compound ball and socket joint; the head of the
talus is the ball, while the superior surface of the spring ligament and the
posterior surface of the Navicular form the socket. The superior surface of the
spring ligament is lined with articular cartilage.
15.3.4.6 Movements
15.3.4.6.1
Inversion
15.3.4.6.2
Eversion
15.3.4.6.3
Navicular Accessory Motions
15.3.4.6.3.1
Inversion
15.3.4.6.3.1.1
Introduction
15.3.4.6.3.1.1.1
When the foot is inverted with supination and plantarflexion
the Navicular slides on the head of the talus in a plantar direction
15.3.4.6.3.1.1.2
When the foot is everted with pronation and Dorsiflexion the
Navicular slides on the head of the talus in a dorsal direction.
15.3.4.6.3.2
Rotation
15.3.4.6.3.2.1
Turning inward, or supination
15.3.4.6.3.3
Dorsal sliding
15.3.4.6.3.4
Supination
15.3.4.6.3.5
Plantarflexion
15.3.4.6.3.6
Adduction
15.3.4.6.3.7
Eversion
15.3.4.6.3.8
Rotation
15.3.4.6.3.8.1
Turning outward, or pronation, of the foot
15.3.4.6.3.9
Plantar Sliding
15.3.4.6.3.10
Pronation
15.3.4.6.3.11
Dorsiflexion
15.3.4.6.3.12
Abduction
15.3.4.7 Ligaments
15.3.4.7.1
Calcaneonavicular (Spring) Ligament
15.3.4.7.1.1
The spring ligament passes from the anterior and medial
margins of the sustentaculum tali forward to the inferior and inferomedial
aspect of the Navicular. Its superior surface articulates with the underside of
the head of the talus. This ligament maintains apposition of the medial aspects
of the forefoot and Hindfoot and in so doing helps to maintain the normal
arched configuration of the foot. Laxity of the ligament allows a medial
separation between the calcaneus and forefoot, with the forefoot assuming an
abducted position with respect to the Hindfoot.
15.3.4.7.1.2
At the same time, the foot is allowed to “untwist” which
effectively lowers the normal arch of the foot, and the talar head is allowed
to move medially and inferiorly.
15.3.4.7.1.3
The plantar calcaneonavicular ligament is a broad and thick band
of fibers, which connects the anterior margin of the sustentaculum tali of the
calcaneus to the plantar surface of the Navicular. This ligament not only
serves to connect the calcaneus and Navicular, but supports the head of the
talus, forming part of the articular cavity in which it is received. The dorsal
surface of the ligament presents a fibrocartilaginous facet, lined by the
synovial membrane, and upon this a portion of the head of the talus rests. Its
plantar surface is supported by the tendon of the Tibialis posterior; its
medial border is blended with the forepart of the deltoid ligament of the
ankle-joint.
15.3.4.7.1.4
The plantar calcaneonavicular ligament, by supporting the head
of the talus, is principally concerned in maintaining the arch of the foot. When
it yields, the head of the talus is pressed downward, medialward, and forward
by the weight of the body, and the foot becomes flattened, expanded, and turned
lateralward, and exhibits the condition known as flat-foot. This ligament
contains a considerable amount of elastic fibers, so as to give elasticity to
the arch and spring to the foot; hence it is sometimes called the “spring”
ligament. It is supported, on its plantar surface, by the tendon of the
Tibialis posterior, which spreads out at its insertion into a number of
fasciculi, to be attached to most of the tarsal and metatarsal bones. This
prevents undue stretching of the ligament, and is a protection against the
occurrence of flat-foot; hence muscular weakness is, in most cases, the primary
cause of the deformity.
15.3.4.7.2
Bifurcate Ligament (Calcaneonavicular Portion)
15.3.4.7.3
Deltoid Ligament (Tibionavicular Portion)
15.3.4.7.4
Dorsal Talonavicular[13]
15.3.4.8 Nerve Supply
15.3.5
Calcaneocuboid
15.3.5.1 Summary
15.3.5.1.1
This synovial, sellar (saddle) joint between the calcaneus
(convex in a dorsal to plantar direction and concave in a medial to lateral
direction) and the cuboid (reciprocally concave and convex)
15.3.5.1.2
This joint is supported by the Calcaneocuboid (Short Plantar)
Ligament, Long Plantar ligament and the Bifurcate Ligament (Calcaneocuboid
Band).
15.3.5.2 Introduction
15.3.5.2.1
The lateral portion of the transverse tarsal joint is the
calcaneocuboid joint.
15.3.5.2.2
The calcaneocuboid joint is a sellar joint in that the
Calcaneal joint surface is concave superoinferiorly and convex mediolaterally;
the adjoining cuboid surface is reciprocally shaped. This joint is enclosed in
a joint capsule distinct from that of the talocalcaneonavicular joint and
constitutes the lateral half of the transverse tarsal joint.
15.3.5.2.3
The joint capsule is reinforced inferiorly by the strong
plantar calcaneocuboid (short plantar) ligament and the long plantar ligament.
15.3.5.2.4
Dorsally, the joint capsule is reinforced by the
calcaneocuboid band of the bifurcate ligament.
15.3.5.2.5
The lateral portion of the transverse tarsal joint is the
calcaneocuboid joint. The calcaneocuboid joint is a sellar joint in that the calcaneal joint
surface is concave superoinferiorly and convex mediolaterally (see Figs.
15-3 and 15-4); the adjoining cuboid surface is reciprocally shaped. This
joint is enclosed in a joint capsule distinct from that of the talocalcaneonavicular
joint and constitutes the lateral half of the transverse tarsal joint. The
joint capsule is reinforced inferiorly by the strong plantar calcaneocuboid (short
plantar) ligament and the long plantar ligament. The short plantar ligament
runs from the anterior tubercle of the plantar aspect of the calcaneus to the
underside of the cuboid. The long plantar ligament runs from the posterior
tubercles of the calcaneus forward to the bases of the fifth, fourth, third,
and sometimes second metatarsals (see Fig. 15-9). Both of these ligaments support the normal
arched configuration of the foot by helping to maintain a twisted relationship
between the hindfoot and forefoot. Dorsally, the joint capsule is reinforced'
by the calcaneocuboid band of the bifurcate ligament (see Fig. 15-10)
15.3.5.3 Definition
15.3.5.3.1
This joint is formed between the calcaneus and the cuboid
bones.
15.3.5.4 Type of Joint
15.3.5.4.1
Synovial
15.3.5.4.2
Sellar (Saddle)
15.3.5.4.2.1
The articulating surface of the calcaneus is convex in a
dorsal to plantar direction and concave in a medial to lateral direction; the
articulating surface of the cuboid is reciprocally concave and convex.
15.3.5.5 Movements
15.3.5.5.1
15.3.5.6 Ligaments
15.3.5.6.1
Introduction
15.3.5.6.1.1
Both the long and short plantar ligaments support the normal
arched configuration of the foot by helping to maintain a twisted relationship
between the Hindfoot and forefoot.
15.3.5.6.2
Calcaneocuboid (Short Plantar) Ligament
15.3.5.6.2.1
The short plantar ligament runs from the anterior tubercle of the
plantar aspect of the calcaneus to the underside of the cuboid.
15.3.5.6.2.2
The plantar calcaneocuboid ligament lies nearer to the bones
than the preceding, from which it is separated by a little Areolar
(ă-realer) tissue. It is a short but wide band of great strength, and
extends from the tubercle and the depression in front of it, on the forepart of
the plantar surface of the calcaneus, to the plantar surface of the cuboid
behind the peroneal groove.
15.3.5.6.3
Long Plantar Ligament
15.3.5.6.3.1
The long plantar ligament runs from the posterior tubercles of
the calcaneus forward to the bases of the fifth, fourth, third, and sometimes
second metatarsals.
15.3.5.6.3.2
The rough surface in front of the calcaneus processes gives
attachment to the long plantar ligament. On the distal end it attaches to the
cuboid bone whose plantar surface presents in front with a deep groove
(Peroneal Sulcus), which runs obliquely forward and medial ward (Peroneus
Longus tendon inserts). Behind this groove is a prominent ridge where the long
plantar ligament is attached.
15.3.5.6.3.3
The long plantar ligament is the longest of all the ligaments
of the tarsus: it is attached behind to the plantar surface of the calcaneus in
front of the tuberosity, and in front to the tuberosity on the plantar surface
of the cuboid bone, the more superficial fibers being continued forward to the
bases of the (sometimes second), third, fourth and fifth metatarsal bones. This
ligament converts the groove on the plantar surface of the cuboid into a canal
for the tendon of the Peroneus longus.
15.3.5.6.4
Bifurcate Ligament (Calcaneocuboid Band)
15.3.6
Metatarsophalangeal Joints (MP)
15.3.6.1 Summary
15.3.6.1.1
This synovial, Condyloid (KON-di-loyd) joint is formed between
the distal ends of the metatarsals with the adjacent end of the proximal
phalanges and supported by the medial and lateral collateral ligaments and the
plantar ligaments. The joint movements are flexion, extension, abduction, and
adduction.
15.3.6.2 Illustration
15.3.6.3 Introduction
15.3.6.3.1
The metatarsophalangeal joints are condyloid formed by the
articulation of the distal ends of the metatarsals with the adjacent ends of
the proximal phalanges.
15.3.6.3.2
The plantar surface of this joint is considerably thickened
because of the plantar ligament at the MP joint and thus flexion is restricted.
15.3.6.3.3
The dorsal surface of the MP joint is loose which permits a
wider rage of extension when compared to flexion.
15.3.6.3.4
Two sesamoid bones are embedded in the plantar ligament of the
big toe.
15.3.6.3.5
The plantar aponeurosis is inserted into slips on either side
of the MP joint.
15.3.6.4 Definition
15.3.6.4.1
This joint is formed between the distal end of the metatarsal
with the adjacent end of the proximal phalanges
15.3.6.5 Type of Joint
15.3.6.5.1
Synovial
15.3.6.5.2
Ellipsoidal or Condyloid (KON-di-loyd)
15.3.6.6 Movements
15.3.6.6.1
Introduction
15.3.6.6.1.1
Flexion and extension are movements about a coronal axis.
15.3.6.6.1.2
Adduction and abduction are movements about a Sagittal axis.
The line of reference for adduction and abduction of the toes is the axial line
projected distally in line with the second metatarsal and extending through the
second digit.
15.3.6.6.1.3
Because abduction of the toes is restricted by the wearing of
shoes, this movement is markedly limited in most adults.
15.3.6.6.2
Flexion 30ş
15.3.6.6.2.1
Movement in a caudal direction
15.3.6.6.3
Extension 40ş
15.3.6.6.3.1
Movement in a cranial direction
15.3.6.6.4
Adduction
15.3.6.6.4.1
Movement toward the axial line
15.3.6.6.5
Abduction
15.3.6.6.5.1
Movement away from the axial line
15.3.6.7 Ligaments
15.3.6.7.1
Medial and Lateral Collateral Ligaments
15.3.6.7.2
Plantar Ligament
15.3.6.8
Nerve Supply
15.3.7
Interphalangeal Joints of Toes (IP)
15.3.7.1 Summary
15.3.7.1.1
This synovial, hinge joint is formed between the adjacent
surfaces of the phalanges and its movements are flexion and extension.
15.3.7.2 Illustration
15.3.7.3 Introduction
15.3.7.3.1
The Interphalangeal joints are ginglymus or hinge joints
formed by the articulations of adjacent surfaces of phalanges
15.3.7.4 Definition
15.3.7.4.1
This joint is formed between the adjacent surfaces of the
phalanges
15.3.7.5 Type of Joint
15.3.7.5.1
Synovial
15.3.7.5.2
Ginglymus (JIN-gli-mus) or Hinge Joints
15.3.7.6 Movements
15.3.7.6.1
Introduction
15.3.7.6.1.1
Flexion and extension are movements about a coronal axis
15.3.7.6.2
Flexion
15.3.7.6.2.1
Movement in a caudal direction
15.3.7.6.3
Extension
15.3.7.6.3.1
Movement in a cranial direction
15.3.7.7
Nerve Supply
15.3.8
Intercuneiform and Cuneocuboid
Articulations
15.3.8.1 Illustration Definition
15.3.8.2 Summary
15.3.8.2.1
These synovial gliding joints are formed between the cuneiform
bones and between the cuneiform and cuboid bones. The joints permit minimal
gliding movements and are supported by dorsal, plantar and Interosseous
Ligaments.
15.3.8.3 Illustrations
15.3.8.4 Definition
15.3.8.4.1
This joint is formed between the adjacent surfaces of the
cuneiform bones
15.3.8.5 Type of Joint
15.3.8.5.1
Synovial
15.3.8.5.2
Gliding
15.3.8.6 Movements
15.3.8.6.1
Minimal Gliding
15.3.8.7 Ligaments
15.3.8.7.1
Dorsal Ligaments
15.3.8.7.1.1
Consist of three transverse bands: one connects the first with
the second cuneiform, another the second with the third cuneiform, and another
the third cuneiform with the cuboid.
15.3.8.7.2
Plantar Ligaments
15.3.8.7.2.1
Similar arrangement to the dorsal, and are strengthened by
slips from the tendon of the Tibialis posterior.
15.3.8.7.3
Interosseous Ligaments
15.3.8.7.3.1
Consist of strong transverse fibers, which pass between the
rough non-articular portions of the adjacent surfaces of the bones.
15.3.9
Tarsometatarsal Articulations
15.3.9.1 Illustration Definition
15.3.9.2 Summary
15.3.9.2.1
These synovial gliding joints are formed between the
cuneiform, cuboid and bases of the five metatarsal bones. These joints permit
minimal gliding movement and are supported by dorsal, plantar and Interosseous
Ligaments.
15.3.9.3 Definition
15.3.9.3.1
This joint is formed between the adjacent surfaces of the
first, second, and third cuneiforms, and the cuboid, which articulate with the
bases of the metatarsal bones.
15.3.9.3.2
The first metatarsal bone articulates with the first
cuneiform; the second is deeply wedged in between the first and third
cuneiforms articulating by its base with the second cuneiform; the third
articulates with the third cuneiform; the fourth, with the cuboid and third
cuneiform; and the fifth, with the cuboid.
15.3.9.4 Type of Joint
15.3.9.4.1
Synovial
15.3.9.4.2
Gliding
15.3.9.5 Movements
15.3.9.5.1
Minimal Gliding
15.3.9.6 Ligaments
15.3.9.6.1
Dorsal Ligaments
15.3.9.6.1.1
The dorsal ligaments are strong, flat bands. The first
metatarsal is joined to the first cuneiform by a broad, thin band; the second
has three, one from each cuneiform bone; the third has one from the third
cuneiform; the fourth has one from the third cuneiform and one from the cuboid;
and the fifth, one from the cuboid.
15.3.9.6.2
Plantar Ligaments
15.3.9.6.2.1
The plantar ligaments consist of longitudinal and oblique bands,
disposed with less regularity than the dorsal ligaments. Those for the first
and second metatarsals are the strongest; the second and third metatarsals are
joined by oblique bands to the first cuneiform; the fourth and fifth
metatarsals are connected by a few fibers to the cuboid
15.3.9.6.3
Interosseous Ligaments
15.3.9.6.3.1
The interosseous ligaments are three in number. The first is
the strongest, and passes from the lateral surface of the first cuneiform to
the adjacent angle of the second metatarsal. The second connects the third
cuneiform with the adjacent angle of the second metatarsal. The third connects
the lateral angle of the third cuneiform with the adjacent side of the base of
the third metatarsal.
15.3.10
Intermetatarsal Articulations
15.3.10.1 Illustration
Definition
15.3.10.2 Summary
15.3.10.2.1
These synovial gliding joints are formed between the adjacent
surfaces of the heads and bases of the five metatarsal bones. The joints permit
minimal gliding movement and are supported by dorsal, plantar, Interosseous
Ligaments and Transverse Metatarsal Ligaments.
15.3.10.3 Definition
15.3.10.3.1
This joint is formed between the adjacent surfaces of the
heads and bases of the five metatarsal bones.
15.3.10.4 Type
of Joint
15.3.10.4.1
Synovial
15.3.10.4.2
Gliding
15.3.10.5 Movements
15.3.10.5.1
Minimal Gliding
15.3.10.6 Ligaments
15.3.10.6.1
Introduction
15.3.10.6.1.1
The base of the first metatarsal is not connected with that of
the second by any ligaments; in this respect the great toe resembles the thumb.
15.3.10.6.1.2
The bases of the other four metatarsals are connected by the
dorsal, plantar, and interosseous ligaments.
15.3.10.6.2
Dorsal Ligaments
15.3.10.6.2.1
Pass transversely between the dorsal surfaces of the bases of
the adjacent metatarsal bones.
15.3.10.6.3
Plantar Ligaments
15.3.10.6.3.1
Similar arrangement to the dorsal
15.3.10.6.4
Interosseous Ligaments
15.3.10.6.4.1
Consist of strong transverse fibers, which connect the rough
non-articular portions of the adjacent surfaces.
15.3.10.6.5
Transverse Metatarsal Ligament
15.3.10.6.5.1
The transverse metatarsal ligament is a narrow band which runs
across and connects together the heads of all the metatarsal bones; it is
blended anteriorly with the plantar (glenoid) ligaments of the
metatarsophalangeal articulations. Its plantar surface is concave where the
Flexor tendons run below it; above it the tendons of the Interossei pass to
their insertions. It differs from the transverse metacarpal ligament in that it
connects the metatarsal to the others.
15.3.11
Synovial Membranes of Foot (Review)
15.3.11.1 Summary
15.3.11.1.1
There are nine synovial membranes in the foot enclosing both
single and multiple articulations in the foot. 1.) Subtalar/Talocalcaneal 2.) Talocalcaneal
3.) Talocalcaneonavicular 4.) Calcaneocuboid 5.) Cuneonavicular 6.)
Intercuneiform, Cuneocuboid, and cuneiform (2nd & 3rd)/metatarsal
(2nd, 3rd, & 4th) 7.) 1st
cuneiform with great toe 8.) Cuboid & Metatarsal (4th & 5th)
9.) Navicular & Cuboid (Rare)
15.3.11.2 Synovial
Membranes
15.3.11.2.1
Subtalar/Talocalcaneal (ta’’lo-kal-ka-ne-al)
15.3.11.2.2
Talocalcaneal
15.3.11.2.3
Talocalcaneonavicular
15.3.11.2.4
Calcaneocuboid
15.3.11.2.5
Cuneonavicular
15.3.11.2.6
Intercuneiform, Cuneocuboid and the articulations of the
second and third cuneiforms with the bases of the second and third metatarsal
bones, and the adjacent surfaces of the bases of the second, third, and fourth
metatarsal bones
15.3.11.2.7
First cuneiform with the metatarsal bone of the great toe
15.3.11.2.8
Cuboid with the fourth and fifth metatarsal bones
15.3.11.2.9
Contiguous surfaces of the Navicular and cuboid bones (Rare)
15.4 Biomechanics (Kessler)
15.4.1
Introduction
15.4.1.1 Summary
15.4.1.1.1
Problems in the biomechanics of the foot ankle complex are
likely to cause abnormal stresses to the joints of the foot and ankle, as well
as to the other weight-bearing joints such as the knee, hip, or lower
spine. The foot/ankle as a movable
pivot helps in the maintenance of balance and economy of energy consumption,
which are adversely affected by inadequate mobility, and improper structural alignment
of the foot ankle complex.
15.4.1.2 The joints of the foot and ankle constitute the first movable pivots
in the weight-bearing extremity once the foot becomes fixed to the ground.
15.4.1.3 Considered together, these joints must permit mobility in all planes
in order to allow for minimal displacement of a person’s center of gravity with
respect to the base of support when walking over flat or uneven surfaces. In
this sense maintenance of balance and economy of energy consumption are, in
part, dependent on proper functioning of the ankle-foot complex.
15.4.1.4 Adequate mobility and proper structural alignment of these joints
are also necessary for normal attenuation of forces transmitted from the ground
to the weight-bearing extremity.
15.4.1.5 Deviations in alignment and changes in mobility are likely to cause
abnormal stresses to the joints of the foot and ankle, as well as to the other
weight-bearing joints. It follows that detection of biomechanical alterations
in the ankle-foot region is often necessary for adequate interpretation of
painful conditions affecting the foot and ankle, as well as conditions
affecting the knee, hip, or lower spine.
15.4.1.6 Figure 15-13
15.4.1.6.1
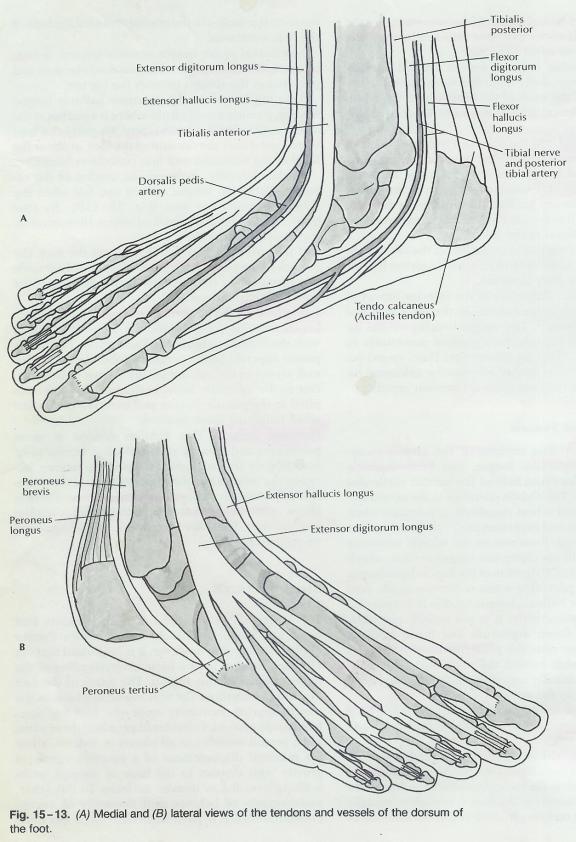
15.4.2
Structural Alignment
15.4.2.1 Summary
15.4.2.1.1
In the normal standing position, the patella faces straight
forward, the knee joint axis lies in the frontal plane and the tibial tubercle
is in line with the midline or lateral half of the patella and a line passing
through the tips of the of the malleoli should be 20° to 25° with the frontal
plane. The lateral Malleolus is positioned inferiorly with respect to the
medial Malleolus such that the intermalleolar line makes an angle of about 10°
with the transverse plane. With the patellae facing straight forward, the feet
should be pointed outward about 5° to 10°. A Valgus positioning of the
calcaneus on the talus is associated with pronation at the Subtalar joint,
whereas a varus hind-foot involves supination. When considering the structure
of the foot as a whole, it is helpful to compare it to a twisted plate; the
calcaneus, at one end, is positioned vertically when contacting the ground, whereas
the metatarsal heads are positioned horizontally when making contact with a
flat surface. The medial arch is dependent almost entirely on the twisted
configuration of the foot, which is maintained statically by the short and long
plantar ligaments and dynamically by the anterior and posterior tibialis
muscles. Referring back to the cardboard model, notice that when the cardboard
is allowed to untwist by inclining the vertical end in one direction and
keeping the other end flat on the table the arch flattens. In the foot,
inclination of the vertical component of the structure, the calcaneus, will
result in similar untwisting or twisting; this results in a respective decrease
or increase in the arching of the foot, if the metatarsal heads remain in
contact with the ground. The person who stands with the heel in a valgus
position will have a relatively "flat" or untwisted foot, whereas a
person whose heel is in a varus position when standing will appear to have a
"high" arch because of increased twisting between hindfoot and
forefoot.
15.4.2.2 In the normal standing position, the patella faces straight forward,
the knee joint axis lies in the frontal plane and the tibial tubercle is in
line with the midline or lateral half of the patella. In this position, a line
passing between the tips of the malleoli should make an angle of about 20° to
25° with the frontal plane. This represents the normal amount of tibial
torsion; the distal end of the tibia is rotated outward with respect to the
proximal end.
15.4.2.3 The lateral Malleolus is positioned inferiorly with respect to the
medial Malleolus such that the intermalleolar line makes an angle of about 10°
with the transverse plane. The joint axis of the ankle mortise joint
corresponds approximately to the intermalleolar line.
15.4.2.4 With the patellae facing straight forward, the feet should be
pointed outward about 5° to 10°.
15.4.2.5 If, when the feet are in normal standing alignment, the patellae
face inward, increased femoral anterversion, increased external tibial torsion,
or both, may be present. Clinically, the fault can be differentiated by
assessing rotational range of motion of the hips and estimating the degree of
tibial torsion by noting the rotational alignment of the malleoli with respect
to the patellae and tibial tubercles.
15.4.2.6 In the presence of increased hip anterversion, the total range of
hip motion will be normal but skewed such that internal rotation is excessive
and external rotation is restricted proportionally. Similar considerations
holds for a situation in which the patellae face outward when the feet are
normally aligned; femoral retroversion, internal tibial torsion, or both, are
likely to exist.
15.4.2.7 With respect to the frontal plane, normal knee alignment may vary
from slight genu valgum to some degree of genu Varum. Since in most persons the
medial femoral condyle extends farther distally than the later condyle, slight
genu valgum tends to be more prevalent.
15.4.2.8 At the hndfoot, the calcaneus should be positioned in vertical
alignment with the tibia. A valgus or varus heel can usually be observed as a
bowing of the Achilles tendon. A Valgus positioning of the calcaneus on the
talus is associated with pronation at the Subtalar joint, whereas a varus
hind-foot involves supination.
15.4.2.9 When considering the structure of the foot as a whole, it is helpful
to compare it to a twisted plate (Figure 15-14); the calcaneus, at one end, is
positioned vertically when contacting the ground, whereas the metatarsal heads
are positioned horizontally when making contact with a flat surface.
15.4.2.10 Figure
15-14
15.4.2.10.1
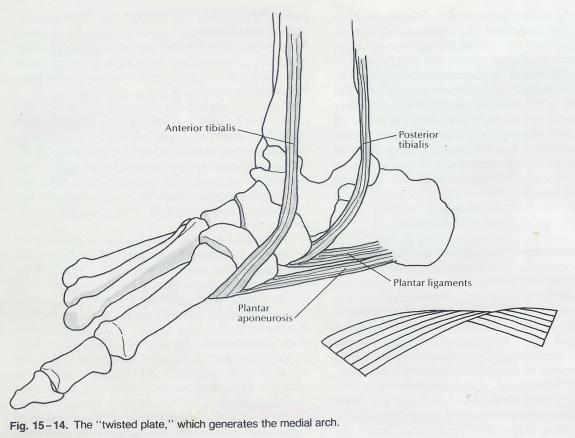
15.4.2.11 Thus,
in the normal standing position on a flat, level surface, the metatarsal heads
are twisted 90° with respect to the calcaneus.
15.4.2.12 To
demonstrate this, a model can be constructed by taking a light rectangular
piece of cardboard and twisting it so that one end lies flat on a table and the
opposite end is perpendicular to the tabletop. Note the “arching” of the
cardboard. This is analogous t the arching of the human foot. It should be
realized that the term arch her applies to the configuration of the structure,
which is dependent on the fact that it is twisted on itself. It does not refer
to an arch in the true architectural sense, in which the arched configuration
is dependent on the shapes of the component “building blocks.”
15.4.2.13 In
the foot, both situations exist. On the medial side, there is little
architectural arching and, therefore, little inherent stability of the medial
arch. The medial arch is dependent almost entirely on the twisted configuration
of the foot, which is maintained statically by the short and long plantar
ligaments and dynamically by the anterior and posterior tibialis muscles. In
contrast, the lateral side of the foot represents a true architectural arch.
(Fig. 15-15) Here the cuboid, being wedged between the calcaneus and
metatarsals, serves as the structural keystone. Only a small component of the
lateral arch is a result of the twisted configuration of the foot.
15.4.2.14 Figure
15-15
15.4.2.14.1
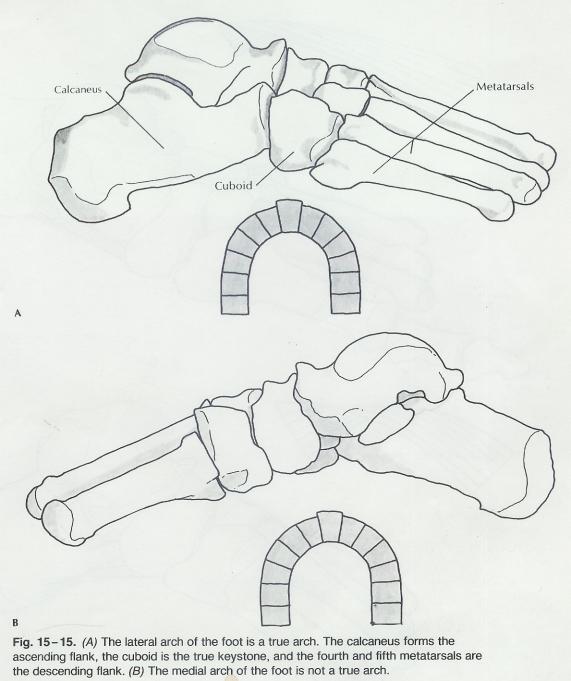
15.4.2.15 Referring
back to the cardboard model, notice that when the cardboard is allowed to
untwist by inclining the vertical end in one direction and keeping the other
end flat on the table the arch flattens. Inclining the vertical end in the
opposite direction increases the twist and increases the arch. In the foot,
inclination of the vertical component of the structure, the calcaneus, will
result in similar untwisting or twisting; this results in a respective decrease
or increase in the arching of the foot, if the metatarsal heads remain in
contact with the ground. (Fig. 15-16)
15.4.2.16 Figure
15-16
15.4.2.16.1
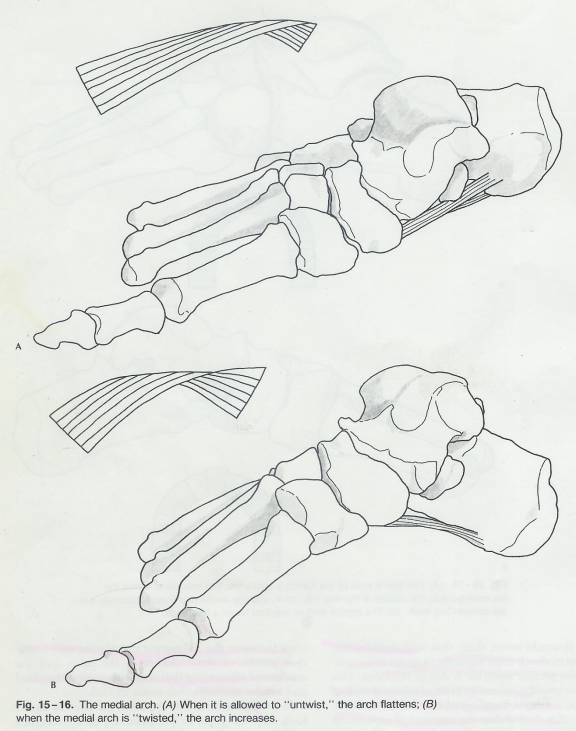
15.4.2.17 The person who stands with the heel in a
valgus position will have a relatively “flat” or untwisted foot, whereas a
person whose heel is in a varus position when standing will appear to have a
“high” arch because of increased twisting between hindfoot and forefoot. The
former situation is often termed a pronated foot or flatfoot, while the latter
is termed a supinated foot or pes cavus. In the situation of the heel remaining
in a vertical position but the metatarsal heads inclined on, say, an uneven
surface, the effect will also be to twist or untwist the foot and, therefore,
to raise or to lower the arch. For example, if the inclination is such that the
first metatarsal head is on a higher level than the fifth, the forefoot
supinates on the hndfoot, untwisting the foot and lowering the arch.
15.4.2.18 Note
that supination of the forefoot with the hindfoot fixed is the same as
pronation of the hindfoot with the forefoot fixed; they both involve untwisting
of the tarsal skeleton from motion at the Subtalar, transverse tarsal, and
tarsal-metatarsal joints.
15.4.2.19 Reference
is often made to a transverse arch of the foot, distinguishing it from the
longitudinal arch. The cardboard model should help to make it clear that some
transverse arching results from the twisted configuration of the foot. This is
simply a transverse component of the arch discussed previously. This transverse
component will increase and decrease along with twisting and untwisting of the
foot. There is also a structural component to the transverse arching of the
foot, resulting from the contours and relationships of the tarsals and
metatarsals. It must be realized, however, that at the level of the metatarsal
heads in the standing subject, not transverse arch exists, since each of the
heads makes contact with the floor.
15.4.2.20 Figure
15-21
15.4.2.20.1
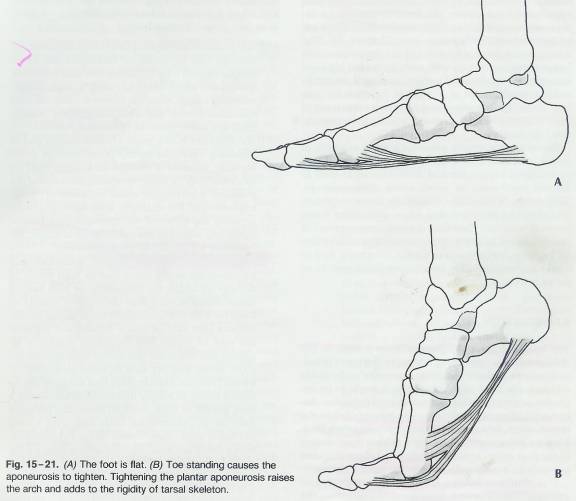
15.4.2.21 Figure
15-22
15.4.2.21.1
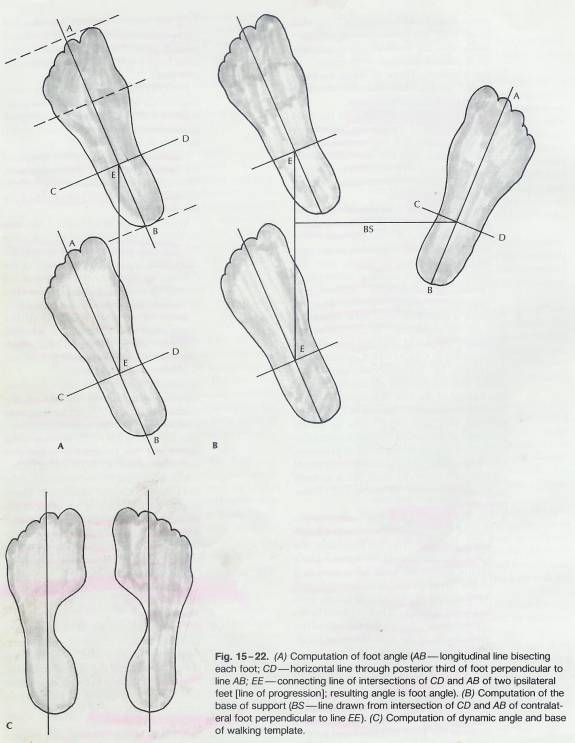
15.4.2.22 Figure
15-23&24
15.4.2.22.1
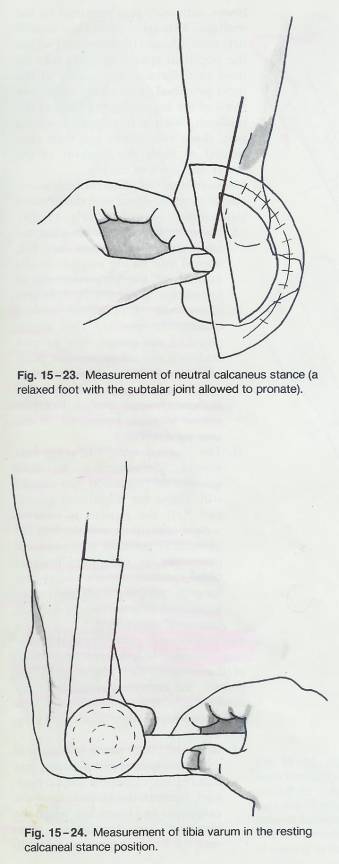
15.4.2.23 Figure
15-25
15.4.2.23.1
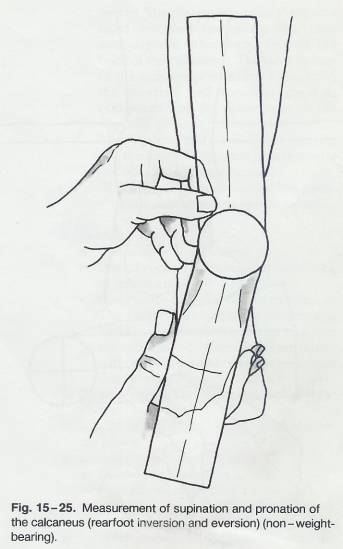
15.4.2.24 Figure
15-26
15.4.2.24.1
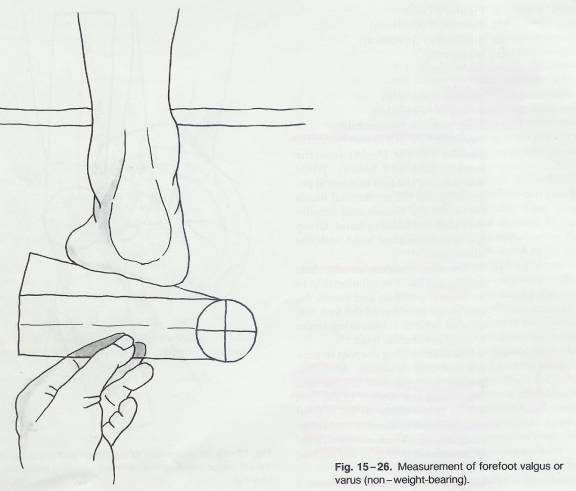
15.4.3
Arthrokinematics of the Ankle-Foot Complex
15.4.3.1 Ankle Mortise Joint
15.4.3.1.1
Summary
15.4.3.1.1.1
The anterior talus is 6mm wider than the posterior talus yet dorsiflexion
does not separate the tibia and fibula more than 2 mm if at all. Both the
anterior and posterior aspects of the talus are in a close, fit with tibial and
fibular malleoli. How does such a wide anterior bone fit into a smaller
posterior articular space? It is because the axis of talar motion is more
medial than lateral, the lateral talar articulation is longer than the medial
articulation, which means it moves a greater distance than the medial talus.
The lateral talus rotates in a helical movement and there is movement of
combined rolling and sliding which also changes the axis of movement
positionally. Thus the final close packed position of full dorsiflexion results
in only minimal tibular/fibular displacement.
15.4.3.1.2
The superior articular surface of the talus is wider
anteriorly than posteriorly, the difference in widths being as much as 6 mm.
The articular surfaces of the tibial and fibular malleoli maintain a close fit
against the medial and lateral articular surfaces of the talus in all positions
of plantar flexion and Dorsiflexion. As the foot moves from full plantar
flexion into full Dorsiflexion the talus rolls backward in the mortise. It
would seem, then, that with ankle Dorsiflexion the malleoli must separate in
order to accommodate the greater anterior width of the talus. This separation
could occur as a result of a lateral shift of the fibula, a lateral bending of
the fibula, or both. However, it is found that the amount of separation that
occurs between the malleoli during ankle dorsiflexion varies from none to only
2 mm, which is much less than would be expected considering the amount of
wedging of the superior articular surface of the talus.
15.4.3.1.3
There appears to be a significant discrepancy between the
difference in anterior and posterior widths of the trochlea of the talus and
the amount of separation that occurs between the tibial and fibular malleoli
with ankle dorsiflexion.
15.4.3.1.4
In understanding this apparent paradox, a closer look must be
given to the structure of the trochlea and the type of movement the talus
undergoes during ankle dorsiflexion. If both sides of the trochlea are examined
it is evident that the lateral articular surface, which articulates with the
fibular Malleolus, is longer in its anteroposterior dimension than the medial articular
surface. The reason for this is that the lateral Malleolus moves over a greater
excursion, with respect to the talus, during plantar flexion-dorsiflexion, than
does the medial Malleolus. This is partly because the axis of motion is farther
from the superior trochlear articular surface laterally than medially. The
corollary to this (and this is true of essentially all joints with sellar
surfaces) is that the relatively trackbound movement that the talus undergoes
on plantar flexion-dorsiflexion at the ankle is not a pure swing, but rather an
impure swing; it involves an element of spin, or rotation, that results in a
helical movement.
15.4.3.1.5
Another way of conceptualizing this movement is to consider
the talus as a section of a cone whose apex is situated medially rotating
within the mortise about its own long axis, rather than a truly cylindrical
body undergoing a simple rolling movement within the mortise. (Figure 15-17)
15.4.3.2 Figure 15-17
15.4.3.2.1
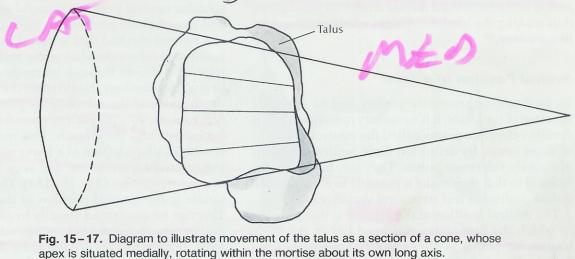
15.4.3.2.2
As a result of this, the intermalleolar lines projected onto
the superior trochlear articular surface at various positions of plantar
flexion and dorsiflexion are not parallel lines. Therefore, the degree of
wedging of the trochlea does not reflect the relative intermalleolar distances
in dorsiflexion and plantar flexion of the ankle. The true intermalleolar
distances are represented by the length of these nonparallel lines projected
onto the superior trochlear surface. The projected line with the foot in
plantar flexion is only slightly shorter, if at all, than that for dorsiflexion
and the necessary separation of the malleoli during dorsiflexion is minimal.
15.4.3.2.3
Up to this point, the ankle mortise joint axis has been
considered as a fixed axis of motion. This has been done for the sake of
simplicity and convenience using the approximate center of movement as the
joint axis. No joint moves about a stationary joint axis. As indicated by
instant center analysis of knee joint motion, this is true of the ankle mortise
joint as well. Their surface velocities determined from the instant centers of
movement show that when moving from full plantar flexion to full dorsiflexion
there is initially a momentary distraction of the Tibiotalar joint surfaces,
followed by a movement of combined rolling and sliding throughout most of the
range, and terminating with an approximation of joint surfaces at the position
of extreme dorsiflexion. These findings are consistent with the fact that the
close packed position of the ankle mortise joint is dorsiflexion; the
tightening of the joint capsule that occurs with movement of any joint into its
close-packed position produces an approximation of the joint surfaces.
15.4.3.3 Subtalar Joint (Talocalcaneal)
15.4.3.3.1
Summary
15.4.3.3.1.1
From the outset, movement at this joint is somewhat difficult
to conceptualize because the posterior articulation between the talus and
calcaneus is concave superiorly on convex inferiorly, while the Anteromedial
articulation is convex on concave. The posterior Calcaneal facet moving against
the opposing concave talar surface can be compared with the radial head moving
within the radial notch of the ulna. In at least some persons, this type of
movement at the Subtalar joint is accompanied by a slight forward displacement
of the talus during pronation and a backward displacement on supination, thus
making the total movement a helical, or screw like motion.
15.4.3.3.2
As discussed previously, this is a compound joint with two
distinct articulations. From the outset, movement at this joint is somewhat
difficult to conceptualize because the posterior articulation between the talus
and calcaneus is concave superiorly on convex inferiorly, while the
Anteromedial articulation is convex on concave. Understanding talocalcaneal
movement is perhaps facilitated by considering it analogous to movement at the
proximal and distal Radioulnar joints. The Radioulnar joints, like the
talocalcaneal joints, move in conjunction with one another and have only one
degree of freedom of motion. The posterior Calcaneal facet moving against the
opposing concave talar surface can be compared with the radial head moving
within the radial notch of the ulna. As this movement occurs the Anteromedial
facet of the talus must move in relation to the concave Anteromedial surface of the calcaneus, just
as the head of the ulna must move within the ulnar notch of the radius at the
distal joint of the forearm. In at least some persons, this type of movement at
the Subtalar join t is accompanied by a slight forward displacement of the
talus during pronation and a backward displacement on supination, thus making
the total movement a helical, or screw like motion.
15.4.3.4 Neutral Position of a Joint
15.4.3.4.1
Summary
15.4.3.4.1.1
The neutral position of the first ray is that position in
which the first metatarsal head lies in the same transverse plane as the
central three metatarsal heads when they are at their most dorsiflexed
position. Root and co-workers describe a neutral position of the Subtalar joint
that is that position of the joint in which the foot is neither pronated nor
supinated. From this position, full supination of the normal Subtalar inverts
the calcaneus twice as many degrees as full pronation everts it. When the
Subtalar joint is held in its neutral position there is no longer the ability
for the midtarsal joint to pronate. According to James, the talar head in a
pronated foot can be palpated as a medial bulge; in a supinated foot the talar
head bulges laterally. The neutral position is usually present when the
longitudinal axis of the lower limb and the vertical axis o f the calcaneus are
parallel. A second method that is useful in the open-chain position involves
visualizing and feeling the Subtalar joint as it moves through its range of
motion. To begin, the examiner should place the ulnar surface of the thumb into
the Sulcus of the patient's foot from pronation to supination and back again.
This can be confirmed visually by observing the lateral curves above and below
the Malleolus.
15.4.3.4.2
To facilitate arthrometric studies and to correlate function
of the joints, it is necessary to assign to joints certain reference points
called the neutral positions of those joints. By definition these neutral
positions are purely reference points. They are, however, significant in that
they make it possible to measure and define positional and structural
variances.
15.4.3.4.3
The neutral position of the first ray is that position in
which the first metatarsal head lies in the same transverse plane as the
central three metatarsal heads when they are at their most dorsiflexed
position. From this neutral position, the first metatarsal can move an equal
distance above and below the transverse plane of the lesser metatarsal heads
when the first ray is moved through its full range.
15.4.3.4.4
Root and co-workers describe a neutral position of the
Subtalar joint that is that position of the joint in which the foot is neither
pronated nor supinated. Another way to state this is the position from which
the Subtalar joint could be maximally pronated and supinated. From this
position, full supination of the normal
Subtalar inverts the calcaneus twice as many degrees as full pronation everts
it. Subtalar neutral is two thirds from inversion and one third from eversion
of the calcaneus.
15.4.3.4.5
Clinically this is important since the Subtalar neutral
position provides a foundation for meaningful and valid measurements and
observation s to take place with respect to the foot and entire leg. It is not
only a basis for meaningful communication but is also the foundation for the
application of precise therapy, such as the fabrication of an effective
biomechanical orthotic device. There is also a direct clinical correlation
between the Subtalar joint and the midtarsal joint. When the Subtalar joint is
held in its neutral position there is no longer the ability for the midtarsal
joint to pronate. The Midtarsal is unable to dorsiflex, evert, or abduct even
the Subtalar is in its neutral position. This position is termed the Normal
locking position of the midtarsal joint.
15.4.3.4.6
According to James, the talar head in a pronated foot can be
palpated as a medial bulge; in a supinated foot the talar head bulges
laterally. In the neutral position the talar head can be palpated equally on
the medial and lateral aspects of the ankle. The neutral position is usually
present when the longitudinal axis of the lower limb and the vertical axis o f
the calcaneus are parallel. This method for establishing the Subtalar neutral
position is useful in both the open and closed-chain positions.
15.4.3.4.7
A second method that is useful in the open-chain position
involves visualizing and feeling the Subtalar joint as it moves through its
range of motion. To begin, the examiner should place the ulnar surface of the
thumb into the Sulcus of the patient’s foot from pronation to supination and
back again. This movement is very similar in shape to that of a horse saddle,
being very abrupt toward pronation and very flat and shallow toward supination
(Fig. 15-18a). The bottom of this saddle is the neutral position of the
Subtalar joint. This can be confirmed visually by observing the lateral curves
above and below the Malleolus (Fig. 15-18b). If these curves are the same
depth, this is the accurate neutral position. If the curve below the Malleolus
is deeper or shallower, then the foot is still in a pronated or supinated
position and should be repositioned.
15.4.3.5 Figure 15-18
15.4.3.5.1
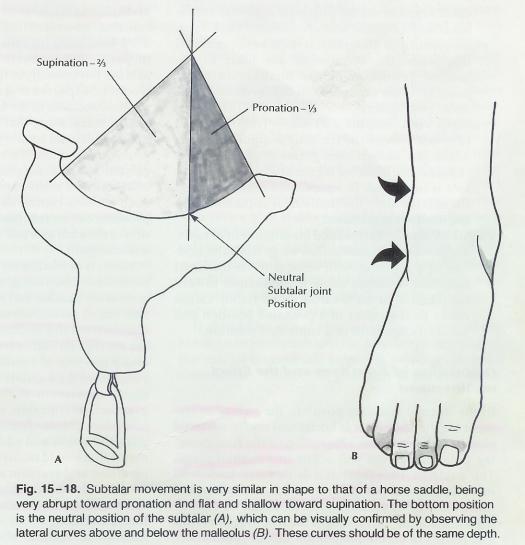
15.4.3.6 Transverse Tarsal Joints: Talonavicular and Calcaneocuboid Joints
15.4.3.6.1
Summary
15.4.3.6.1.1
Although the talonavicular joint is a ball and socket joint
with potential multidirectional movement because the navicular is closely bound
to the cuboid bone laterally, the movement allowed at the calcaneocuboid joint
largely governs its freedom of movement. The axis of motion of most concern is
the axis of pronation and supination. Such an orientation allows a movement of
inversion-adduction-plantar flexion (supination) and eversion-abduction-dorsiflexion
(pronation) of the forefoot. In the standing position, movement and positioning
of the transverse tarsal joint occurs in conjunction with subtalar joint
movement; when the subtalar joint pronates, the transverse tarsal joint supinates
and vice versa.
15.4.3.6.2
Although perhaps some movement occurs between the cuboid and
navicular, movement of these two bones is considered here as a unit with
respect to the calcaneus and the talus.
The configuration of the talonavicular articulation is essentially that
of a ball and socket joint. Because of
this configuration, it potentially has three degrees of freedom of movement,
allowing it to move in all planes.
However because the navicular is closely bound to the cuboid bone
laterally, its freedom of movement is largely governed by the movement allowed
at the calcaneocuboid joint. The calcaneocuboid joint, having a sellar
configuration, has two degrees of freedom, each of which occurs about a
distinct access of motion.
15.4.3.6.3
The axis of motion of most concern is the axis of pronation
and supination. This axis is similar in
location and orientation to the subtalar joint axis, the major difference being
that it is not inclined as much vertically.
It passes through the talar head, backward, downward, and laterally. Such an orientation allows a movement of
inversion-adduction-plantar flexion (supination) and
eversion-abduction-dorsiflexion (pronation) of the forefoot. In the standing position, movement and
positioning of the transverse tarsal joint occurs in conjunction with subtalar
joint movement; when the subtalar joint pronates, the transverse tarsal joint
supinates and vice versa. Pronation of the forefoot close packs and locks the
transverse tarsal joint complex, whereas supination results in loose packing
and a greater degree of freedom of movement
15.4.3.7 Osteokinematics of the Ankle-Foot Complex
15.4.3.7.1
Terminology
15.4.3.7.1.1
At this point, some terms related to movements and positioning
of various components of the ankle and foot must be clarified.
15.4.3.7.1.2
Inversion-Eversion
15.4.3.7.1.2.1
Movement about a horizontal axis lying in the Sagittal plane.
Functionally pure inversion and eversion rarely occur at any of the joints of
ankle or foot. More often they occur as a component of supination or pronation.
15.4.3.7.1.3
Abduction-Adduction
15.4.3.7.1.3.1
Movement of the forefoot about a vertical axis or the movement
of the forefoot that results from internal or external rotation of the hindfoot
with respect to the leg.
15.4.3.7.1.4
Internal-External Rotation
15.4.3.7.1.4.1
Movement between the leg and hindfoot occurring about a
vertical axis. Pure rotations do not occur functionally but rather occur as
components of pronation and supination.
15.4.3.7.1.5
Plantar flexion-Dorsiflexion
15.4.3.7.1.5.1
Movement about a horizontal axis lying in the plane
corresponding to the intermalleolar line. Functionally, these usually occur in
conjunction with other movements.
15.4.3.7.1.6
Pronation-Supination
15.4.3.7.1.6.1
Functional movements occurring around the obliquely situated
subtalar or transverse tarsal joint axis. At both of these joints, pronation
involves abduction, eversion and some dorsiflexion; supination involves adduction,
inversion, and plantar flexion of the distal segment on the proximal segment.
This is because these joint axes are inclined backward, downward, and
laterally. It must be appreciated that when the metatarsals are fixed to the
ground, pronation of the hindfoot (subtalar joint) involves supination of the
forefoot (transverse tarsal joint).
15.4.3.7.1.7
Pronated Foot-Supinated Foot
15.4.3.7.1.7.1
Traditionally, a pronated foot (in the standing position) is
one in which the arched configuration of the foot is reduced; the hindfoot is
pronated while the forefoot is supinated. In a supinated foot (standing) the
arch is high, the hindfoot is supinated, and the forefoot is pronated.
15.4.3.7.1.8
Valgus-Varus
15.4.3.7.1.8.1
Terms used for alignment of parts. Valgus denotes inclination
away from the midline of a segment with respect to its proximal neighbor,
whereas varus is inclination toward the midline. At the hindfoot and forefoot,
valgus refers to alignment in a pronated position and varus to alignment in a
supinated position.
15.4.3.8 Orientation of Joint Axes and the Effect on Movement
15.4.3.8.1
Summary
15.4.3.8.1.1
In the normal standing position, the axis of movement for the
knee joint is horizontal and in a frontal plane. The ankle mortise joint axis
is directed backward mediolaterally about 25° from the frontal plane and
downward from medial to lateral about 10° to 15° from horizontal. Movement of
the free foot about this axis results in combined plantar flexion, adduction,
and inversion or combined dorsiflexion, abduction, and eversion. Note that the
above statements relate the movements at the respective joints to the
orientations of the joint axes when the foot and leg are swing feely. The
obvious question in this regard would be; How is it possible to move both the
tibia and femur in the Sagittal plane such as when performing a knee bend with
the knee pointed forward, when movement is occurring at the ankle and knee
about two nonparallel axes? Since the knee joint axis lies horizontally in the
frontal plane, no problem would be expected there, since it is ideally oriented
to allow rotation of the bones in the Sagittal plane. It would seem, then, that
by performing such a knee bend an internal rotatory movement must be applied to
the ankle, since the ankle joint axis I externally rotated with respect to the
frontal plane. This apparent problem can be resolved by considering the
orientation of the joint axis and associated movements at the subtalar joint.
The average deviation from the midline of the foot is 23°, whereas the average
deviation from the horizontal is 42°. Because the axis of motion for the
subtalar joint deviates from the Sagittal plane and from the horizontal plane,
movement at this joint involves combined eversion, dorsiflexion, and abduction
or combined inversion, plantar flexion, and adduction. Subtalar movement is
essentially uniaxial, so that any movement occurring at the joint may occur
only in conjunction with its component movements, that is, abduction can only
occur in conjunction with eversion and dorsiflexion the three together
constituting pronation at the subtalar. Supination causes the calcaneus to
assume a varus position, which, since the metatarsals remain flat on the
ground, increases the twist in the foot and raises the arch.
15.4.3.8.2
In the normal standing position, the axis of movement for the
knee joint is horizontal and in a frontal plane. With flexion and extension of
the free-swinging tibia, movement will occur in a Sagittal plane. The ankle
mortise joint axis is directed backward mediolaterally about 25° from the
frontal plane and downward from medial to lateral about 10° to 15° from
horizontal. Movement of the free foot about this axis results in combined
plantar flexion, adduction, and inversion or combined dorsiflexion, abduction,
and eversion. Note that the above statements relate the movements at the
respective joints to the orientations of the joint axes when the foot and leg
are swing feely. In this position, movements at one joint may occur
independently of the other.
15.4.3.8.3
Therapists must be more concerned, however, with what happens
when the foot becomes fixed to the ground and movement occurs simultaneously at
the joints of the lower extremities. This is the situation during
weight-bearing activities, or normal functional activities, involving the leg.
The obvious question in this regard would be; How is it possible to move both
the tibia and femur in the Sagittal plane such as when performing a knee bend
with the knee pointed forward, when movement is occurring at the ankle and knee
about two nonparallel axes? The heavy-weight lifter largely avoids the problem
by pointing the knees outward, thus using external rotation and abduction at
the hip.
15.4.3.8.4
This brings the knee and ankle axes closer to parallel
alignment. The fact remains, however, that it is possible to perform a deep
knee bend with the knee directed forward, and through a considerable range.
Since the knee joint axis lies horizontally in the frontal plane, no problem
would be expected there, since it is ideally oriented to allow rotation of the
bones in the Sagittal plane. It would seem, then, that by performing such a
knee bend an internal rotatory movement must be applied to the ankle, since the
ankle joint axis I externally rotated with respect to the frontal plane. Surely
ankle mortise joints cannot be expected to withstand such stresses during daily
activities. This apparent problem can be resolved by considering the
orientation of the joint axis and associated movements at the subtalar joint.
15.4.3.8.5
The axis of motion for the subtalar joint is directed backward,
downward, and laterally. The degree of inclination and mediolateral deviation
of the axis varies greatly among persons. The average deviation from the
midline of the foot is 23°, whereas the average deviation from the horizontal
is 42°. Because the axis of motion for the subtalar joint deviates from the
Sagittal plane and from the horizontal plane, movement at this joint involves
combined eversion, dorsiflexion, and abduction or combined inversion, plantar
flexion, and adduction. Note that pure abduction and adduction of the foot are
movements that would occur about a vertical axis and that inversion and
eversion occur about a purely horizontal axis. Since the subtalar axis is
positioned about midway between horizontal and vertical, it follows that
movement about this axis would include elements of adduction-abduction as well
as eversion-inversion.
15.4.3.8.6
Now again consider a situation of simultaneous knee and ankle
flexion in the Sagittal plane with the foot fixed (deep knee bend). It was
indicated that with such a movement an internal rotatory moment of the tibia on
the talus would be applied to the ankle. Because the subtalar axis allows an
element of movement about a vertical axis (rotation in the horizontal plane),
this internal rotatory moment can be transmitted to the subtalar joint.
Internal rotation of the tibia on the foot is, of course, equivalent to
external rotation of the foot on the leg, which is referred to as abduction of
the foot. Subtalar movement is essentially uniaxial, so that any movement
occurring at the joint may occur only in conjunction with its component
movements, that is, abduction can only occur in conjunction with eversion and
dorsiflexion the three together constituting pronation at the subtalar. Thus
with the foot fixed, simultaneous dorsiflexion of the ankle and flexion of the
knee, keeping the leg in the Sagittal plane, requires pronation at the subtalar
joint.
15.4.3.8.7
As a corollary to this, with such a movement, if pronation at
the subtalar joint is restricted, and abnormal internal tibial rotatory stress
will occur at the ankle mortise joint, an internal femoral rotatory stress will
be placed on the knee, or both. The need for subtalar movement can be reduced
by moving the leg out of the Sagittal plane (by pointing the knee outward),
bringing the knee and ankle axes into closer alignment.
15.4.3.8.8
Similar considerations apply to the situation of a person
rotating the leg over a fixed foot. Any rotation imparted to the tibia is
transmitted to the subtalar joint (Fig. 15-19).
15.4.3.9 Figure 15-19
15.4.3.9.1
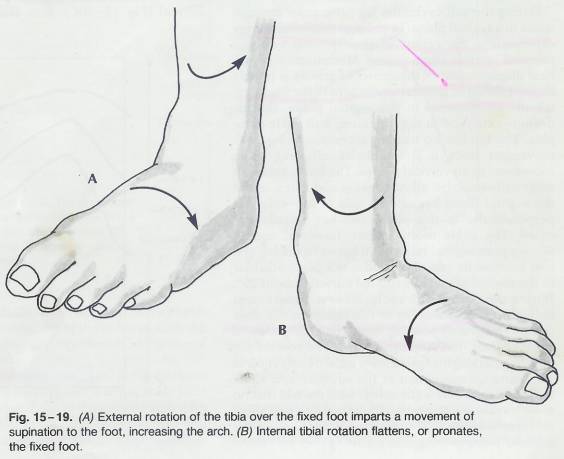
15.4.3.9.2
For example, if on rotates the leg externally over a foot that
is fixed to the ground, the subtalar joint undergoes a movement of supination.
This is analogous to movement about a mitered hinge; movement of one component
about a vertical axis is transmitted to the second component as movement about
a horizontal axis. Supination causes the calcaneus to assume a varus position,
which, since the metatarsals remain flat on the ground, increases the twist in
the foot and raises the arch. The opposite occurs with internal tibial
rotation; pronation of the hindfoot causes a relative supination of the
forefoot; the foot untwists and the arch flattens. This can easily be observed
if one attempts to rotate the leg with the foot fixed to the ground. With
respect to the structural alignment, then , a person with excessive internal
tibial torsion will tend to have a pronated hindfoot (calcaneus in valgus
position) and a forefoot that is supinated with respect to the hindfoot. The resultant untwisting of the foot causes
a flatfoot on standing. The person with excessive external tibial torsion will
tend to have a varus heel and a high arch.
15.4.3.9.3
The degree of twisting and untwisting of the foot also varies
with stance width. When standing with the feet far apart, the heel tends to
deviate into a valgus position with respect to the floor and the metatarsal
heads remain flat; the metatarsals assume a position of supination with respect
to the heel, thus untwisting the foot. The opposite occurs when standing with
the legs crossed.
15.4.3.9.4
It should be noted that when standing with the hindfoot in
pronation and the forefoot in supination the medial metatarsals assume a
position closer to dorsiflexion. Since the joint axis of the first metatarsal
is obliquely oriented (from Anterolateral to Posteromedial), dorsiflexion of
the first metatarsal involves a component of abduction away from the midline of
the foot.
15.4.3.9.5
Therefore, in a pronated foot, the first metatarsal is usually
positioned in varus. On the other hand, a person with metatarsus primus varus,
a condition in which the first metatarsal deviates into varus position, the
foot will tend to assume a pronated position. This is because in order for the
first metatarsal to be in a varus angle, it must also be in some dorsiflexion.
This causes supination of the forefoot, which necessitates pronation of the
hindfoot in order for the person to stand with the metatarsal head and
calcaneus in contact with the ground.
15.4.3.10 Ankle
and Foot During Gait
15.4.3.10.1
Summary
15.4.3.10.1.1
Clinicians must be concerned with the function of the joints
of the ankle and foot during normal daily activities. The prime consideration
here, of course, is walking. Again, because these are weight-bearing joints and
because the foot becomes fixed to the ground during the stance phase, an
understanding of the biomechanical interrelationships between these joints and
the other joints of the lower extremity is necessary. During the gait cycle,
the leg progresses through space in a Sagittal plane. Minimization of vertical
displacement of the center of gravity is largely accomplished by angular
movement of the lower extremity components in the Sagittal plane, that is,
flexion-extension at the hip, knee, and ankle complex. The hip has no trouble
accommodating such movement since it is multiaxial, allowing some movement in
all vertical planes. The knee, although essentially uniaxial, allows flexion
and extension in the Sagittal plane because its axis of movement is
perpendicular to this plane and horizontally oriented. Movement of the tibia in
a parasagittal plane over a fixed foot requires simultaneous movement at the
subtalar and ankle mortise joints. Those muscles that affect movement at the
ankle mortise joint also cross the subtalar joint, moving it as well. During
the first interval, each of the segments of the lower extremity rotates
internally with respect to its more proximal neighboring segment; the pelvis
rotates internally in space, the femur rotates internally on the pelvis, and
the tibia rotates internally on the femur. Much of this internal rotation is
absorbed at the subtalar joint as pronation. Internal rotation of the leg with
respect to the foot, occurring at the subtalar joint, makes the axis o the
ankle mortise joint more perpendicular to the plane of progression. This is
because the obliquity of the ankle axis, in the coronal plane, imposes a
component of adduction of the foot during plantar flexion. At heel-strike, a
moment-arm equal to the distance between the point of heel contact and the
ankle joint develops. This results in some flexion at the knee, which is
consistent with the fact that the tibia is rotating internally with respect to
the femur. Through the early period of foot-flat, during which most of
dorsiflexion occurs, the segments of the lower extremity continue to rotate
internally. This occurs as supination at the subtalar joint. Consistent with
this, the Peroneus longus muscle contracts, maintaining a pronated twist of the
metatarsals and bring the foot as a whole toward its twisted
configuration.Internal rotation of the talus with respect to the calcaneus
occurs as pronation, while external rotation results in supination at the
subtalar joint. Also, once the foot becomes flat on the ground, the metatarsal
heads become fixed and the twisting and untwisting of the foot becomes dependent
on the position of the hind foot. A relatively common condition that
illustrates the biomechanical interdependency of the weight-bearing joints is
femoral anteversion.
15.4.3.10.1.2
15.4.3.10.2
Clinicians must be concerned with the function of the joints
of the ankle and foot during normal daily activities. The prime consideration
here, of course, is walking. Again, because these are weight-bearing joints and
because the foot becomes fixed to the ground during the stance phase, an
understanding of the biomechanical interrelationships between these joints and
the other joints of the lower extremity is necessary.
15.4.3.10.3
During the gait cycle, the leg progresses through space in a
Sagittal plane. In order to minimize energy expenditure, the center of gravity
must undergo minimal vertical displacement. Minimization of vertical
displacement of the center of gravity is largely accomplished by angular
movement of the lower extremity components in the Sagittal plane, that is,
flexion-extension at the hip, knee, and ankle complex. The hip has no trouble
accommodating such movement since it is multiaxial, allowing some movement in
all vertical planes. The knee, although essentially uniaxial, allows flexion
and extension in the Sagittal plane because its axis of movement is
perpendicular to this plane and horizontally oriented. The ankle mortise join,
however, cannot allow a pure Sagittal movement between the leg and foot because
its axis of motion is not perpendicular t the Sagittal plane; it is rotated
outward about 25°.During the normal gait cycle, however, movement between the
foot and leg in the Sagittal plane does occur. This is only possible through
participation of another joint, the subtalar joint. Movement of the tibia in a
parasagittal plane over a fixed foot requires simultaneous movement at the
subtalar and ankle mortise joints. This is consistent with the fact that no
muscles attach to the talus. Those muscles that affect movement at the ankle
mortise joint also cross the subtalar joint, moving it as well.
15.4.3.10.4
In considering the various movements occurring at each of the
segments of the lower extremity during gait, it is convenient to speak of three
intervals of stance phase; these are 1.) The interval from heel-strike to
foot-flat 2.) Midstance (foot-flat), and 3.) The interval from the beginning of
heel-rise to toe-off.
15.4.3.10.5
During the first interval, each of the segments of the lower
extremity rotates internally with respect to its more proximal neighboring
segment; the pelvis rotates internally in space, the femur rotates internally
on the pelvis, and the tibia rotates internally on the femur. (Fig. 15-20a)
15.4.3.11 Figure
15-20
15.4.3.11.1
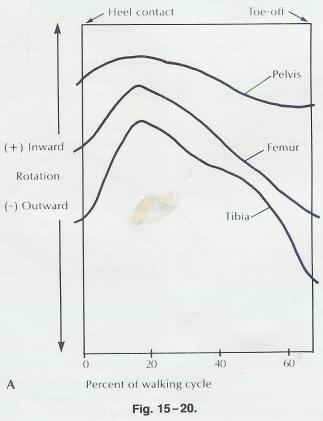
15.4.3.11.2
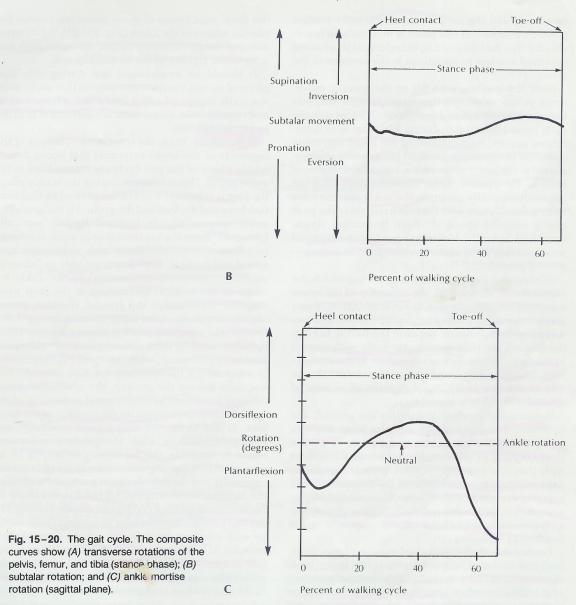
15.4.3.11.3
15.4.3.11.4
It follows that the entire lower limb rotates during this
phase and that distal segments rotate more, in space, than the more proximally situated
segments. At the point of heel strike, the foot becomes partially fixed to the
ground, so that only minimal internal torsion between the heel and the ground
takes place. Much of this internal rotation is absorbed at the subtalar joint
as pronation (Fig. 15-20b). Internal rotation of the leg with respect to the
foot, occurring at the subtalar joint, makes the axis of the ankle mortise
joint more perpendicular to the plane of progression.
15.4.3.11.5
This allows the ankle mortise joint to provide for movement in
the Sagittal plane, which, of course, is the plantar flexion occurring at the
ankle during this interval (Fig. 15-20c). Note that the foot tends to deviate
slightly medially in this stage of stance phase. This is because the obliquity
of the ankle axis, in the coronal plane, imposes a component of adduction of
the foot during plantar flexion.
15.4.3.11.6
At heel-strike, a moment-arm equal to the distance between the
point of heel contact and the ankle joint develops. The reactive force of the
ground acting on the foot at heel-strike across this moment-arm will tend to
swing the tibia forward in the Sagittal plane. This results in some flexion at
the knee, which is consistent with the fact that the tibia is rotating
internally with respect to the femur. Knee flexion, form an extended position,
involves a component of internal tibial rotation
15.4.3.11.7
Note that at heel-strike, the hindfoot moves into pronation
while the tibialis anterior muscle contracts to bring the forefoot into
supination. This causes the foot to untwist, the transverse tarsal joint to
unlock, and the joints to assume a loose packed position. The foot at this
point is in a position favorable for free mobility and is, therefore, at its
greatest potential to adapt to variations in the contour of the ground.
15.4.3.11.8
Once the foot becomes flat on the ground, movement at the
ankle mortise joint changes abruptly from plantar flexion to dorsiflexion.
Through the early period of foot-flat, during which most of dorsiflexion
occurs, the segments of the lower extremity continue to rotate internally. This
rotation is transmitted to the joints of the ankle, since the foot is now fixed
to the ground. Some internal rotation of the tibia automatically occurs at the
ankle mortise joint during dorsiflexion with the foot fixed, since the joint
axis is inclined about 15° from horizontal, downward and laterally. However,
most of the internal rotation takes place at the subtalar joint as a component
of pronation.
15.4.3.11.9
Throughout most of the period during which the foot is flat on
the ground, the segments of the lower extremity rotate externally; the more
distal segments rotate externally to a greater degree than their proximal
neighbors. Again, because the foot is fixed to the ground, the tibia rotates
externally with respect to the foot. This occurs as supination at the subtalar
joint. The change during foot-flat, from internal rotation to external
rotation, takes place after most of ankle dorsiflexion is complete.
15.4.3.11.10
Because the forefoot is fixed to the ground, the inversion occurring
at the subtalar joint imposes pronation at the transverse tarsal joint, causing
a close-packing or locking of the tarsus. Consistent with this, the Peroneus
longus muscle contracts, maintaining a pronated twist of the metatarsals and
bring the foot as a whole toward its twisted configuration. The foot at this
point is being converted into an intrinsically stable lever capable of
providing for the trust of pushing off.
15.4.3.11.11
During the final interval of stance phase, from heel-rise to
toe-off, the segments of the lower limb continue to rotate externally. This
external rotation of the tibia is again transmitted to the subtalar joint as
supination of the hindfoot. With contraction of the calf muscle, the ankle
begins plantar flexion, creating a thrust for push-off. This again creates a
moment arm acting on the knee, this time moving the tibia into extension with
respect to the femur. Note that this is consistent with the external rotation
of the tibia occurring during this phase, since knee extension involves a
component of external tibial rotation.
15.4.3.11.12
The extension of the metatarsophalangeal joints, occurring
with heel-rise, causes a tightening of the plantar aponeurosis through a
windlass effect, since the distal attachment of the aponeurosis crosses the
plantar aspect of the joints. This tightening of the aponeurosis further raises
the arch and adds to the rigidity of the tarsal skeleton.
15.4.3.11.13
It should be emphasized that during this final phase of
stance, the joint movements that occur automatically convert the foot into a
stable lever system and require little, if any, muscle contraction in order to
accomplish this.
15.4.3.11.14
In summary, then, the transverse rotations of the segments of
the lower extremity that occur during stance phase of the gait cycle are
transmitted to the ankle joints. This is because during the stance phase the
foot is relatively fixed to the ground so that rotation between the foot and
the ground is minimal. The ankle mortise axis Is inclined slightly vertically
(about 15° from the horizontal axis) while the subtalar joint axis is situated
about midway between horizontal and vertical. Both joints are able to “absorb”
rotatory movements transmitted to the ankle because of the vertical inclination
of the joint axes. With the foot fixed to the ground, the tibia rotates
slightly internally at the ankle mortise during dorsiflexion and externally
during plantar flexion. Internal rotation of the talus with respect to the
calcaneus occurs as pronation, while external rotation results in supination at
the subtalar joint. Also, once the foot becomes flat on the ground, the
metatarsal heads become fixed and the twisting and untwisting of the foot
becomes dependent on the position of the hind foot. At heel-strike, the tibia
rotates internally, pronating the hindfoot, while the tibialis anterior
contracts to supinate the forefoot; this results in an untwisted foot. During
midstance, as the tibia rotates externally and the subtalar joint gradually
supinates, the foot becomes twisted. This twisting is further increased by
tightening of the plantar aponeurosis. The twisted configuration results in
maximal joint stability with minimal participation fo the intrinsic foot
muscles. It is the twisted foot, then, that is best suited for weight-bearing
and propulsion. During midstance there is little activity of the intrinsic
muscles in the normal foot because the twisted configuration confers a passive,
or intrinsic, stability on the foot. However, in the flatfooted person,
considerably more muscle action is required since the foot is relatively
untwisted. The flatfooted person must rely more on extrinsic stabilization by
the muscles. An untwisted configuration is desirable in situations in which the
foot must be mobile, such as in adapting to surface contours of the ground. Consistent
with this is the fact that at heel-strike, the foot assumes an untwisted state
in preparation for conformation to the contacting surface.
15.4.3.11.15
A relatively common condition that illustrates the
biomechanical interdependency of the weight-bearing joints is femoral
anteversion. A person with femoral anteversion must stand with the leg
internally rotated in order to position the hip joint in normal (neutral)
alignment. Conversely, if the leg is positioned in normal alignment with the
patella facing straight forward, the hip joint assumes a position of relative
external rotation. External rotation at the hip decreases the congruity of the
joint surfaces.
15.4.3.11.16
During stance phase, as the femur externally rotates on the
pelvis, the hip of the person with femoral anteversion will tend to go into too
much external rotation. As the slack is taken up in the part of the joint
capsule that pulls tight on external rotation, the joint receptors sense the
excessive movement. To avoid excessive joint incongruity and to prevent abnormal
stress to the joint capsule, the person with femoral anteversion must
internally rotate during stance phase. This internal rotation is transmitted
primarily to the subtalar joint, which is best oriented to accommodate
transverse rotations. internal rotation at the subtalar joint causes pronation
of the hindfoot; the foot untwists and the arch flattens. Thus, femoral
anterversion predisposes to pronation of the foot. Also, because of the
internal rotatory movement transmitted through the knee, femoral anteversion
may also be a causative factor in certain knee disorders. For example, internal
rotation imposed on a semiflexed knee causes the knee to assume an increased
valgus position. This may predispose to patellar tracking dysfunction. Since
there is a tendency for the person with femoral anteversion to walk with
increased hip joint incongruity, the force of weight bearing is transmitted to
a smaller area of contact at the articular surface of the hip. The result may
be accelerated wear of the hip joint surfaces, perhaps leading to degenerative
hip disease.
15.4.3.11.17
Femoral anteversion provides a good example of how a
structural abnormality in one region may lead to localized biomechanical
disturbances as well as altered mechanics at joints some distance away. This,
again, is especially true of the lower extremity and should emphasize that when
evaluating many patients with foot disorders it may be appropriate, if not
necessary, to examine the structure and function of the knee, hip, and lower
back. Conversely, foot or ankle dysfunction may precipitate disturbance in more
proximal joint.
15.5 START
15.6 Examination
15.6.1
15.7
Ankle
Joint
Kendall 22
Back Table of Contents References
15.7.1.1 Type of Joint
15.7.1.1.1
15.7.1.2 Movements Permitted
15.7.1.2.1
15.7.1.3 Range of Movement
15.7.1.3.1
15.7.1.3.2
15.7.1.4 Description
15.7.1.4.1
15.7.1.5 Flexion and Extension
15.7.1.5.1
Flexion
15.7.1.5.2
15.7.1.5.3
Extension
15.7.1.5.4
15.7.1.6 Confusion
15.7.1.6.1
15.7.1.7 Measurement
15.7.1.7.1
Back Table of Contents References
15.8.1.1 Type of Joint
15.8.1.1.1
15.8.1.2 Movements Permitted
15.8.1.2.1
15.8.1.3 Range of Movement
15.8.1.3.1
15.8.1.3.2
15.8.1.4 Description
15.8.1.4.1
15.8.1.5 Talonavicular Joint
15.8.1.5.1
Supination and Pronation
15.8.1.5.1.1
15.8.1.5.1.2
Supination
15.8.1.5.1.2.1
15.8.1.5.1.3
Pronation
15.8.1.5.1.3.1
15.8.1.6 Transverse Tarsal Joints
15.8.1.6.1
Adduction and Abduction
15.8.1.6.1.1
15.8.1.6.1.2
Adduction
15.8.1.6.1.2.1
15.8.1.6.1.3
Abduction
15.8.1.6.1.3.1
15.8.1.6.1.4
Inversion
15.8.1.6.1.4.1
15.8.1.6.1.5
Eversion
15.8.1.6.1.5.1
Back Table of Contents References
15.9.1.1 Type of Joint
15.9.1.1.1
15.9.1.2 Movements Permitted
15.9.1.2.1
15.9.1.3 Range of Movement
15.9.1.3.1
15.9.1.4 Description
15.9.1.4.1
15.9.1.5 Flexion and Extension
15.9.1.5.1
15.9.1.5.2
Flexion
15.9.1.5.3
15.9.1.5.4
Extension
15.9.1.5.5
15.9.1.6 Adduction and Abduction
15.9.1.6.1
15.9.1.6.2
Adduction
15.9.1.6.3
15.9.1.6.4
Abduction
15.9.1.6.5
Back Table of Contents References
15.10.1.1
Type of Joint
15.10.1.1.1
15.10.1.2
Movements Permitted
15.10.1.2.1
15.10.1.3
Range of Movement
15.10.1.3.1
15.10.1.3.2
15.10.1.4
Description
15.10.1.4.1
15.10.1.5
Flexion and Extension
15.10.1.5.1
15.10.1.5.2
Flexion
15.10.1.5.3
15.10.1.5.4
Extension
15.10.1.5.5
Back Table of Contents References
17.1.1.1 Type of Joint
17.1.1.1.1
17.1.1.2 Movements Permitted
17.1.1.2.1
17.1.1.3 Range of Movement
17.1.1.3.1
17.1.1.3.2
17.1.1.4 Description
17.1.1.4.1
17.1.1.5 Atlanto-occipital Articulation
17.1.1.5.1
17.1.1.6 Convexity
17.1.1.6.1
17.1.1.7 Confusion
17.1.1.7.1
17.1.1.8 Flexion
17.1.1.8.1
17.1.1.9 Extension
17.1.1.9.1
17.1.1.10
Hyperextension
17.1.1.10.1
17.1.1.11
Lateral Flexion
17.1.1.11.1
17.1.1.12
Rotation
17.1.1.12.1